Physiological Mechanisms Underlying High Salt Diets and Cancer
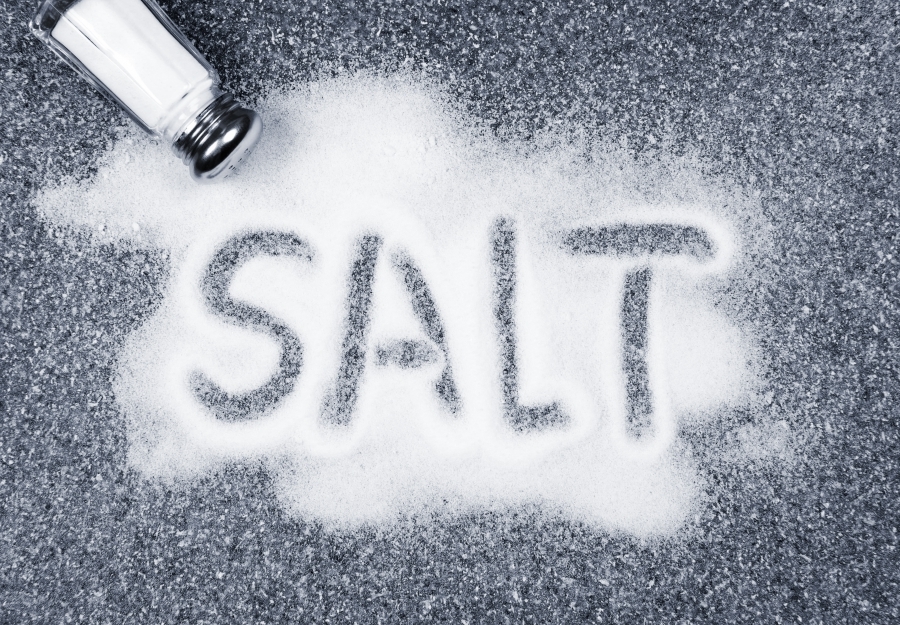
I recently received a question: “I would also like to know what the purported mechanism for low sodium and low cancer is?”
Let me first preface this question with my original blog that precipitated the question. Dr. Jansson sent me this in his unpublished book manuscript on May 10, 1997. Accordingly, the information in his book is now more than 20 years old and unfortunately, Dr. Jansson died on May 23, 1998. Dr. Jansson’s book runs about 300 pages in length, is hand typed, double spaced, and contains the following 10 chapters:
- Background to the Potassium/Sodium Hypothesis
- Human Diet before Modern Times
- Diet and Dietary Carcinogens and Anticarcinogens
- Non-Dietary Carcinogens and Anticarcinogens
- Aging and Cellular and Total-Body Potassium and Sodium
- Hypo- and Hyperkalemic Diseases and Cancer
- Altitude and Cellular Potassium, Sodium, and Cancer
- Animal Experiments
- Earlier Studies of Relations between Potassium, Sodium, and Cancer
- Conclusions, Explanations, and Recommendations
Contained in Dr. Jansson’s book are numerous references that he uses to explain cellular mechanisms of action underlying the epidemiological evidence that he summarizes demonstrating the relationship between dietary sodium (Na+), potassium (K+) and cancer.
Clearly, since I have not obtained permission to publish his book from his heirs, it would not be ethical for me to release it yet. However, I can tell you that many of the cellular mechanisms involving sodium, potassium, cancer initiation and promotion, that Dr. Jansson discusses, were well known 20 years ago by cancer scientists and oncologists. These mechanisms are virtually identical to those same ones that Dr. Jansson had previously described in his scientific publications (1-8).
I would like to point out that Dr. Jansson’s work with sodium, potassium, and cancer was not exclusively unique to his scientific publications or those of his scientific colleagues. Two other well-known cancer scientists, Maryce Jacobs and Roman Pieta independently published an extensive review chapter (9) on the same topic while exhaustively reviewing almost all human studies (epidemiology and case reports), tissue (cell culture) studies, animal studies as well as mechanisms of action.
These authors conclusions were as follows:
Generally, a high potassium to sodium ratio is associated with decreased cancer incidence. Conversely, a higher sodium to potassium ratio with a higher absolute sodium concentration is associated with a higher risk of cancer. (9)
Early Studies on Sodium, Potassium, and Cancer
It has been long recognized that modifications in the cell surface are implicated in the oncogenic and mitogenic processes, including changes in ion transport, membrane permeability, transmembrane potential, and intracellular ion concentrations (3, 6, 9,10-16).
Cone and colleagues have pointed out that the chronic lowering of the transmembrane potential initiates the mitogenic process, and that environmental events which reduce the activity of the Na+/K+ pump in the cell surface membrane initiate and sustain mitosis (10-16). The notion that elevated intracellular Na+ concentrations are increased in cancer cells, whereas K+ concentrations are reduced, has been demonstrated in tissue studies as well as in vivo data from a variety of human and animal experiments (9, 17-20).
One of the challenges in the interpretation of these early experiments was the technology that was employed to measure intracellular Na+, K+ and other ions. Generally, these ions were determined by technologies (flame emission photometry, atomic absorption analysis, electron probe X-ray microanalysis) that necessitated penetration of the cell wall and required correction for the contaminating presence of extracellular fluid ions which introduced large errors in the data (21).
Accordingly, the clues to the etiology of cancer provided by these early ion experiments went largely ignored by later cancer workers who focused upon more complex hormone, cytokine (localized hormones) and immune mediated mechanisms of cancer.
Nevertheless, a new technology (presently called 23NaMRI, previously called 23NaNMR) was developed by FW Cope between 1965-1970 (22-23) which for the first time allowed for accurate intracellular ionic concentration measurements in muscle, brain, and kidney tissues without disrupting cellular membranes and without contaminating the internal cellular ion content with extracellular fluid ions.
Unfortunately, the clout of this powerful new technology went largely unappreciated by cellular physiologists until the early 1980’s when it finally began to gain acceptance as a method for non-invasive and non-destructive determination of intracellular sodium in viable cells and tissue (21, 24).
Only a relative few cellular biologists began to examine intracellular Na concentrations in cancer cells using 23NaMRI (21, 24). Hence, the notion of altered ionic concentrations in cancer cells remained largely unrecognized by most oncologists and cancer scientists. Further, specific studies of salt loading in humans and accurate measurements (23NaMRI) of intracellular Na+ and K+ concentrations in various human tissues would still lie in the future.
Recent Studies on Sodium, Potassium, and Cancer, and Mechanisms of Action
The first study to investigate salt loading and examine intracellular sodium concentrations (via 23NaMRI) in any human body tissue was performed in 1994 by Resnick and colleagues (25) who were interested not in cancer, but in hypertension. In both, hypertensives and normal subjects fed high salt diets (200 meq/day) for two months, significant increases in erythrocyte (red blood cell) intracellular Na+ concentrations occurred (25).
Interestingly, blood plasma concentrations of Na+ are regulated by the kidney within a very narrow range (135-145 mmol/L) that is stable within 2 to 3 mmol/L over 3 year periods in humans (26), and only show transient elevations during increased periods of elevated salt consumption (27, 28).
Accordingly, the prevailing dogma in the scientific literature had been that increased dietary Na+ intakes were acutely (within hours or days) removed from the bloodstream (plasma) and excreted by the kidney into the urine via commensurate increases in water retention. Hence, when dietary Na+ is increased from low to high levels, total body Na+ and water increase until daily Na+ excretion again equals intake.
This dogma had prevailed in the scientific literature for over 50 years but should have started to crumble with the Resnick et al. (25) study (using 23NaMRI data) which indicated that erythrocytes retain Na+ intracellularly when plasma concentrations of Na+ are temporarily elevated.
The implications of the Resnick et al. study (25) are suggestive that Cope’s (22, 23) original hypothesis (22, 23), first formulated in 1965-70, was correct in suggesting that many body tissues (muscle, kidney, brain) tend to retain Na+ ions when plasma concentrations are acutely elevated. Plasma concentrations of Na+ shortly return to normal ranges after dietary intakes are elevated (27, 28), but tissue intracellular concentrations do not (29-32).
Perhaps, the most important insight of these new developments is the recognition that certain body tissues, particularly skin, skeletal muscle, erythrocytes and other tissues (29, 33-38) act as reservoirs to retain Na+ intracellularly, long after transient plasma changes in Na+ concentrations occur.
This important new data implicates dietary salt as being integral in the etiology of autoimmune disease (38, 39 -41), cardiovascular disease (42-45), chronic inflammation (38, 39, 42, 46-57) and now alarmingly in multiple types of cancer (58-67), as previously outlined by Jansson (1-8) Jacobs (9) and others more than 20 years ago.
Mechanisms Underlying Cancer from Salt Ingestion
Cancers generally maintain five developmental stages:
- Initiation
- Promotion
- Malignant conversion
- Invasion
- Metastasis (68).
In virtually every developmental stage of cancer, inflammation plays a crucial role (68-75). Although numerous genetic and environmental factors underlie cancer development and progression, it is clear that elevated dietary salts act at all five developmental stages of cancer to promote and initiate cancer via salt’s promotion of inflammation, tumor-genic growth factors and pro-carcinogenic modulation of the innate and adaptive immune systems.
DNA Damage, Repair and Salt
Cells have evolved mechanisms which constantly monitor DNA for any damage or breaks in its integrity and then proceed to repair the broken or damaged DNA (74, 75). DNA damage occurs during acute inflammation and subsequently accumulates during chronic inflammation. DNA repair mechanisms are important in countering damage to DNA, but are not always successful, often times leading to cancer promotion (74, 75).
Burg and colleagues have demonstrated that a high salt environment increases the number of DNA breaks both in cell cultures and in vivo (76-78). Acute elevations of osmolality from 300 (the normal concentration in plasma) to 500-600 mosmol/kg H2O by adding NaCl causes DNA strand breaks both in tissue and in vivo experiments which persists even after the cells have adapted to higher salt environments (77, 78).
High salt diets can cause tissue (skin, muscle, erythrocyte, brain, kidney) intracellular concentrations of Na+ to increase beyond plasma concentrations of Na+ (29, 33-38). Accordingly, as long as high dietary salt intakes are continued, then the higher concentrations of Na+ found in the tissue reservoirs of Na+ (skin, muscle, erythrocytes, brain and other tissues) will put them at increased risk for DNA damage, inflammation and cancer promotion not only from increased DNA breaks but also from its associated pro-inflammatory milieu (74, 76).
Reactive Oxygen Species (ROS) and Salt
In biological systems ROS (superoxide, hydrogen peroxide, hydroxyl radicals) can be produced intracellularly from exogenous sources (diet, tobacco smoke, pollutants, radiation, drugs, and salt consumption) reacting with cellular contents. ROS are also formed endogenously as a normal product of oxygen metabolism and maintain important roles in cellular signaling and homeostasis. During times of environmental stress (high ultraviolet radiation, pollutants, high salt intake, etc.), ROS concentrations may increase dramatically which can significantly damage cell structures. This process is referred as oxidative stress and produces chronic inflammation (74, 75) which may lead to cancer (79, 80).
Elevations of salt in tissue culture studies as well as in vivo animal models indicate that chronic increases in salt consumption can have deleterious health effects by increasing ROS production (81-88). Increased ROS production in turn acts synergistically to promote DNA damage and a pro-inflammatory microenvironment conducive to cancer formation (74, 79, 80).
Vascular Endothelial Growth Factor (VEGF) and Salt
Angiogenesis, the creation of new vessels from established vasculature is crucial to tumor growth and metastasis. Angiogenesis is regulated by multiple factors, of which VEGF plays a principal role. Various approaches to inhibit the VEGF signaling pathway by directly targeting VEGF itself or by blocking its receptors have been developed and have become established techniques for treating a wide variety of cancers (89-93). VEGF causes angiogenesis in tumors via multiple mechanisms including the activation of specific signaling pathways (NFAT5 and AKT/PI3k) (59, 94).
At least two human studies have demonstrated significant increases in circulating concentrations of VEGF-C following consumption of high salt diets (95, 96). The human data corroborates animal experiments also showing that high salt diets elevate VEGF-C (97-99).
High salt cellular environments have recently been demonstrated to directly induce the expression of VEGF in breast cancer cells via a number of mechanisms including the NFAT5 signaling pathway (59). Studies of breast cancer patients have indicated a strong correlation between the genetic expression of NFAT5 and the development of breast cancer (100). This accumulating information clearly indicates that a high salt environment promotes angiogenesis in tumor cells.
Salt Induced Warburg Metabolism in Cancer Cells
One of the defining characteristics of all cancer cells, unlike normal cells, is that they utilize a seemingly inefficient metabolism of glucose, producing large amounts of lactate as opposed to synthesizing ATP. This abnormal metabolism of glucose by all cancer cells is called aerobic glycolysis and was first identified by Otto Warburg in 1924 (101, 102) and hence, it is called the “Warburg Effect” of cancer cells (60, 101, 102).
Although the exact causes of the “Warburg Effect” of all cancer cells are unclear, high osmotic stress in the solid tumor microenvironment is considered to be one of the most important factors (60, 77, 78). As I have previously shown, a high salt diet transiently increases plasma Na+ concentrations (25, 27) which causes elevated concentrations of salt in almost all of the body’s tissues including skin, muscle, erythrocytes, brain, and kidney (23, 25, 33-38) that creates high osmotic stress (77, 78) in these tissues.
Amara and colleagues have demonstrated that a high salt environment exaggerates the Warburg Effect in breast cancer cells by increasing osmotic stress (60). Accordingly, dietary salt may act to promote cancer cell proliferation in various tissues by enhancing the Warburg Effect in actively growing tumors.
Salt Induced Pro-Cancer Activation of Macrophages
Macrophages are a type of white blood cells that engulf and digest cellular debris, foreign substances, microbes, and cancer cells. A subtype of these cells, called M1 macrophages, encourage inflammation and promotes cancer, whereas those macrophages that decrease inflammation and encourage tissue repair are called M2 macrophages (61, 103-104).
High salt environments activate M1 macrophages to produce a pro-inflammatory M1 state (40, 104) that promotes cancer while simultaneously inducing a M2 environment with poor phagocytic ability to engulf and remove cancer cells (61). Accordingly, the epidemiological evidence indicating that a high salt diet promotes cancer (1-9) is consistent with the molecular physiologic evidence.
Salt and T Cell Activation
A high salt environment not only activates macrophages of the innate immune system to promote cancer, but it also activates CD4+ T helper (Th) cells (46) which form an essential part of the antigen specific, adaptive immune system whose function is governed by micro-environmental cues (105). Local tumor inflammatory conditions cause a number of immune cells, including CD4+ T helper (Th) cells to be recruited to the local tumor site (58).
A high salt environment induces CD4+ T helper (Th) cells to differentiate into Th17 cells (39, 51) which then secrete the pro-inflammatory cytokine (IL-17) within the tumor micro-environment (46, 58). IL-17 induces angiogenesis in the tumor microenvironment by causing increased secretion of VEGF which leads to enhanced tumorigenicity and cancer metastasis (51, 73). Mechanistically, high salt environments promote the activation of CD4+ T helper (Th) cells into Th17 cells and the subsequent secretion of the pro-inflammatory cytokine (IL-17) via the NFAT5, the p38/MAPK, the AKT/PI3k and the SGK1 signaling pathways (39, 51, 59, 94).
High salt diets not only promote cancer via their activating and pro-inflammatory effects upon CD4+ T helper cells and the formation of Th17 cells (46, 58), but also upon their impairment of regulatory T cells (Treg); key elements of the adaptive immune system. Treg cells normally produce anti-inflammatory properties via secretion of the immuno-suppressive IL-10 cytokine (58).
High salt environments have been demonstrated to promote interferon release from Treg cells and abolish their normal anti-inflammatory and immunosuppressive effects (106, 107). Once again, high salt environments appear to adversely affect Treg cells via their effect upon the SGK1 signaling pathway (106). The suppressive effect of high salt environments upon Treg function supports the conclusion from epidemiological studies (1-9) that a high salt, low potassium environment represents a key nutritional factor that promotes and initiates cancer.
Conclusions
The average U.S. diet (per day) contains 3,584 mg of sodium (Na+) and 2,795 mg of potassium (K+) yielding a K+/Na+ ratio of 0.77 (108). This ratio (below 1.00) should fundamentally shake you, as it demonstrates the dysfunction and disease-promoting effects of the typical U.S. diet. Few natural, unsalted foods maintain K+/Na+ ratios less than 1.00.
From my prior post and spreadsheet (109), which extensively examines the sodium (Na+) and potassium (K+) content of more than 1,200 plant and animal foods (unadulterated without added salt), two key conclusions are revealed:
- It is difficult or impossible when eating natural, non-salted, foods to consume more than 2,300 mg of Na+ per day, and
- It is virtually impossible when eating real, non-salted foods to consume less potassium than sodium. Non-salted, natural foods, contain 5 to 10 times more potassium than sodium (109). In the typical U.S. diet, we eat about 23% more sodium than potassium (108).
Consequently, this data provides indisputable information regarding the ancestral nutritional conditions (high potassium, low sodium) that conditioned the human genome, but also the genomes of all terrestrial vertebrates as well as the genomes of all simple celled organisms, as life on earth evolved from unicellular organisms into multicellular organisms including fish, amphibians, terrestrial dwelling reptiles, birds, mammals and hominins (us).
All cells in human, mammalian and terrestrial species have evolved a cellular mechanism called the sodium (Na+)/potassium (K+) ATP-ase pump. The Na+/K+ ATP-ase pump maintains the internal cellular ionic environment at rest by pumping Na+ out of the cell to maintain a low internal cellular Na+ environment, but also to keep the internal cellular potassium environment high (110, 111).
Further, terrestrial and aquatic vertebrates have evolved kidneys which filter blood to remove excessive blood (plasma) stores of Na+, but also serve to maintain high blood concentrations of K+ in blood (plasma) (112, 113). This is the natural physiological state of affairs under which virtually all life on earth evolved – a low intracellular sodium environment and a high potassium intracellular environment (114, 115).
The regular inclusion of mined, artificial salt into the human diet, which has only occurred regularly in the past 10,000 years (333 human generations) (116, 117), represents a fundamental nutritional disruption for which our genomes have no recourse except disease (1-9, 25, 27, 29, 34, 58-67), physiological dysregulation (38, 39, 41, 42, 46-57), and premature aging and senescence (118-121).
The contemporary Paleo Diet is not necessarily about pleasing our modern palettes and penchants for unlimited salt, sugar and refined carbohydrates, but rather it is about emulating the dietary characteristics of our pre-agricultural ancestors (116, 117). A lifetime dietary intake of what is typically perceived as an innocuous salt intake/addiction is actually a high salt/low potassium diet (115). This abnormal nutritional condition (high salt intake/low potassium intake) puts all people at increased risk for most chronic diseases of civilization and premature death.
References
1.Jansson B. Potassium, sodium, and cancer: a review. J Environ Pathol Toxicol Oncol. 1996;15(2-4):65-73
2.Jansson B. Dietary, total body, and intracellular potassium-to-sodium ratios and their influence on cancer. Cancer Detect Prev. 1990;14(5):563-5
3.Jansson B. Intracellular electrolytes and their role in cancer etiology. In Thompson JR, Brown BW, eds. Cancer modeling. New York: Marcel Dekker 1987:1-59.
4.Jansson B. Geographic cancer risk and intracellular potassium/sodium ratios. Cancer Detect Prev. 1986;9(3-4):171-94
5.Jansson B, Jankovic J. Low cancer rates among patients with Parkinson’s disease. Ann Neurol. 1985 May;17(5):505-9
6.Newmark HL, Wargovich MJ, Bruce VR, Boynton AL, Kleine LP, Whitfield JF. Jansson B, Cameron IL. Ions and neoplastic development. In: Mastromarino AJ, Brattain MG, eds. Large bowel cancer. Clinical and basic science research. Cancer Research Monographs, Vol 3, New York: Praeger Publisher 1985:102-129.
7.Jansson B. Geographic mappings of colorectal cancer rates: a retrospect of studies, 1974-1984. Cancer Detect Prev. 1985;8(3):341-8
8.Jansson B. Seneca County, New York: an area with low cancer mortality rates. Cancer. 1981 Dec 1;48(11):2542-6
9.Jacobs MM, Pienta RJ. Relationships between potassium and cancer. In: Vitamins and Minerals in the Prevention and Treatment of Cancer (Jacobs MM [ed.]), 1991, CRC Press, Boston, Chapter 16, 227-245.
10.Cone CD Jr. Electroosmotic interactions accompanying mitosis initiation in sarcoma cells in vitro. Trans N Y Acad Sci. 1969 Apr;31(4):404-27
11.Cone CD Jr. Variation of the transmembrane potential level as a basic mechanism of mitosis control. Oncology. 1970;24(6):438-70
12.Cone CD Jr, Tongier M Jr. Control of somatic cell mitosis by simulated changes in the transmembrane potential level. Oncology. 1971;25(2):168-82
13.Cone CD Jr. Unified theory on the basic mechanism of normal mitotic control and oncogenesis. J Theor Biol. 1971 Jan;30(1):151-81.
14.Cone CD Jr, Tongier M Jr. Contact inhibition of division: involvement of the electrical transmembrane potential. J Cell Physiol. 1973 Dec;82(3):373-86
15.Cone CD Jr, Cone CM. Induction of mitosis in mature neurons in central nervous system by sustained depolarization. Science. 1976 Apr 9;192(4235):155-8
16.Cone CD Jr. Ionically mediated induction of mitogenesis in CNS neurons. Ann N Y Acad Sci. 1980;339:115-31
17.Cameron IL, Smith NK, Pool TB, Sparks RL. Intracellular concentration of sodium and other elements as related to mitogenesis and oncogenesis in vivo. Cancer Res. 1980 May;40(5):1493-500
18.Nagy IZ, Lustyik G, Nagy VZ, Zarándi B, Bertoni-Freddari C. Intracellular Na+:K+ ratios in human cancer cells as revealed by energy dispersive x-ray microanalysis. J Cell Biol. 1981 Sep;90(3):769-77.
19.Ranade SS, Panday VK. Major metals in human cancer: calcium, magnesium, sodium and potassium. Sci Total Environ. 1985 Jan;41(1):79-89.
20.Smith NR, Sparks RL, Pool TB, Cameron IL. Differences in the intracellular concentration of elements in normal and cancerous liver cells as determined by X-ray microanalysis. Cancer Res. 1978 Jul;38(7):1952-9
21.Liebling MS, Gupta RK. A comparison of intracellular sodium ion concentrations in neoplastic and nonneoplastic human tissue using 23Na NMR spectroscopy. Ann N Y Acad Sci. 1987;508:149-63.
22.Cope FW. Nuclear magnetic resonance evidence for complexing of sodium ions in muscle. Proc Natl Acad Sci U S A. 1965 Jul;54(1):225-7
23.Cope FW. Spin-echo nuclear magnetic resonance evidence for complexing of sodium ions in muscle, brain, and kidney. Biophys J. 1970 Sep;10(9):843-58.
24.Hansen LL, Rasmussen J, Friche E, Jaroszewski JW. Method for determination of intracellular sodium in perfused cancer cells by 23Na nuclear magnetic resonance spectroscopy. Anal Biochem. 1993 Nov 1;214(2):506-10.
25.Resnick LM, Gupta RK, DiFabio B, Barbagallo M, Mann S, Marion R, Laragh JH.
Intracellular ionic consequences of dietary salt loading in essential hypertension. Relation to blood pressure and effects of calcium channel blockade. J Clin Invest. 1994 Sep;94(3):1269-76
26.Gao S, Cui X, Wang X, Burg MB, Dmitrieva NI. Cross-sectional positive association of serum lipids and blood pressure with serum sodium within the normal reference range of 135-145 mmol/L. Arterioscler Thromb Vasc Biol. 2017 Mar;37(3):598-606.
27.He FJ, Markandu ND, Sagnella GA, de Wardener HE, MacGregor GA. Plasma sodium: ignored and underestimated. Hypertension. 2005 Jan;45(1):98-102.
28.Suckling RJ, He FJ, Markandu ND, MacGregor GA. Dietary salt influences postprandial plasma sodium concentration and systolic blood pressure. Kidney Int. 2012 Feb;81(4):407-11.
29.Titze J, Rakova N, Kopp C, Dahlmann A, Jantsch J, Luft FC. Balancing wobbles in the body sodium. Nephrol Dial Transplant. 2016 Jul;31(7):1078-81.
30.Titze J. Water-free Na+ retention: interaction with hypertension and tissue hydration. Blood Purif. 2008;26(1):95-9.
31.Titze J. Water-free sodium accumulation. Semin Dial. 2009 May-Jun;22(3):253-5.
32.Heer M, Frings-Meuthen P, Titze J, Boschmann M, Frisch S, Baecker N, Beck L.
Increasing sodium intake from a previous low or high intake affects water, electrolyte and acid-base balance differently. Br J Nutr. 2009 May;101(9):1286-94.
33.Kopp C, Linz P, Wachsmuth L, Dahlmann A, Horbach T, Schöfl C, Renz W, Santoro D, Niendorf T, Müller DN, Neininger M, Cavallaro A, Eckardt KU, Schmieder RE, Luft FC, Uder
M, Titze J. (23) Na magnetic resonance imaging of tissue sodium. Hypertension. 2012 Jan;59(1):167-72
34.Kopp C, Linz P, Dahlmann A, Hammon M, Jantsch J, Müller DN, Schmieder RE, Cavallaro A, Eckardt KU, Uder M, Luft FC, Titze J. 23Na magnetic resonance imaging-determined tissue sodium in healthy subjects and hypertensive patients. Hypertension. 2013 Mar;61(3):635-40
35.Dahlmann A, Dörfelt K, Eicher F et al. Magnetic resonance-determined sodium removal from tissue stores in hemodialysis patients. Kidney Int. 2015 Feb;87(2):434-41.
36.Machnik A, Neuhofer W, Jantsch J, et al. Macrophages regulate salt-dependent volume and blood pressure by a vascular endothelial growth factor-C-dependent buffering mechanism. Nat Med. 2009 May;15(5):545-52.
37.Wiig H, Schröder A, Neuhofer W, Jantsch J, Kopp C et al. Immune cells control skin lymphatic electrolyte homeostasis and blood pressure. J Clin Invest. 2013 Jul;123(7):2803-15
38.Jantsch J, Schatz V, Friedrich D et al. Cutaneous Na+ storage strengthens the antimicrobial barrier function of the skin and boosts macrophage-driven host defense. Cell Metab. 2015 Mar 3;21(3):493-501.
39.Kleinewietfeld M, Manzel A, Titze J, Kvakan H, Yosef N, Linker RA, Muller DN, Hafler DA. Sodium chloride drives autoimmune disease by the induction of pathogenic TH17 cells. Nature. 2013 Apr 25;496(7446):518-22
40.Hucke S, Eschborn M, Liebmann M, Herold M, Freise N, Engbers A, Ehling P, Meuth SG, Roth J, Kuhlmann T, Wiendl H, Klotz L. Sodium chloride promotes pro-inflammatory macrophage polarization thereby aggravating CNS autoimmunity. J Autoimmun. 2016 Feb;67:90-101.
41.Zostawa J, Adamczyk J, Sowa P, Adamczyk-Sowa M. The influence of sodium on pathophysiology of multiple sclerosis. Neurol Sci. 2017 Mar;38(3):389-398.
42.Dmitrieva NI, Burg MB. Elevated sodium and dehydration stimulate inflammatory signaling in endothelial cells and promote atherosclerosis. PLoS One. 2015 Jun 4;10(6): e0128870. doi: 10.1371/journal.pone.0128870.
43.McDonough AA, Veiras LC, Guevara CA, Ralph DL. Cardiovascular benefits associated with higher dietary K+ vs. lower dietary Na+: evidence from population and mechanistic studies. Am J Physiol Endocrinol Metab. 2017 Apr 1;312(4): E348-E356.
44.Mozaffarian D, Fahimi S, Singh GM, Micha R, Khatibzadeh S, Engell RE, Lim S, Danaei G, Ezzati M, Powles J; Global Burden of Diseases Nutrition and Chronic Diseases Expert Group.
Global sodium consumption and death from cardiovascular causes. N Engl J Med. 2014 Aug 14;371(7):624-34
45.He FJ, Li J, Macgregor GA. Effect of longer-term modest salt reduction on blood pressure. Cochrane Database Syst Rev. 2013 Apr 30;(4):CD004937
46.Schatz V, Neubert P, Schröder A, Binger K, Gebhard M, Müller DN, Luft FC, Titze J, Jantsch J. Elementary immunology: Na+ as a regulator of immunity. Pediatr Nephrol. 2017 Feb;32(2):201-210.
47.Hernandez AL, Kitz A, Wu C, Lowther DE, Rodriguez DM, Vudattu N, Deng S, Herold KC, Kuchroo VK, Kleinewietfeld M, Hafler DA. Sodium chloride inhibits the suppressive function of FOXP3+ regulatory T cells. J Clin Invest. 2015 Nov 2;125(11):4212-22.
48.Yi B, Titze J, Rykova M, Feuerecker M, Vassilieva G, Nichiporuk I, Schelling G, Morukov B, Choukèr A. Effects of dietary salt levels on monocytic cells and immune responses in healthy human subjects: a longitudinal study. Transl Res. 2015 Jul;166(1):103-10.
49.Zhou X, Zhang L, Ji WJ, Yuan F, Guo ZZ, Pang B, Luo T, Liu X, Zhang WC, Jiang TM, Zhang Z, Li YM. Variation in dietary salt intake induces coordinated dynamics of monocyte subsets and monocyte-platelet aggregates in humans: implications in end organ inflammation. PLoS One. 2013 Apr 4;8(4):e60332.
50.Zhou X, Yuan F, Ji WJ, Guo ZZ, Zhang L, Lu RY, Liu X, Liu HM, Zhang WC, Jiang TM, Zhang Z, Li YM. High-salt intake induced visceral adipose tissue hypoxia and its association with circulating monocyte subsets in humans. Obesity (Silver Spring). 2014 Jun;22(6):1470-6.
51.Wu C, Yosef N, Thalhamer T, Zhu C, Xiao S, Kishi Y, Regev A, Kuchroo VK. Induction of pathogenic TH17 cells by inducible salt-sensing kinase SGK1. Nature. 2013 Apr 25;496(7446):513-7.
52.Kostyk AG, Dahl KM, Wynes MW, Whittaker LA, Weiss DJ, Loi R, Riches DW. Regulation of chemokine expression by NaCl occurs independently of cystic fibrosis transmembrane conductance regulator in macrophages. Am J Pathol. 2006 Jul;169(1):12-20.
53.Lang KS, Fillon S, Schneider D, Rammensee HG, Lang F. Stimulation of TNF alpha expression by hyperosmotic stress. Pflugers Arch. 2002 Mar;443(5-6):798-803.
54.Ip WK, Medzhitov R. Macrophages monitor tissue osmolarity and induce inflammatory response through NLRP3 and NLRC4 inflammasome activation. Nat Commun. 2015 May 11;6:6931.
55.Foss JD, Kirabo A, Harrison DG. Do high-salt microenvironments drive hypertensive inflammation? Am J Physiol Regul Integr Comp Physiol. 2017 Jan 1;312(1):R1-R4
56.Binger KJ, Gebhardt M, Heinig M et al. High salt reduces the activation of IL-4- and IL-13-stimulated macrophages. J Clin Invest. 2015 Nov 2;125(11):4223-38
57.Min B, Fairchild RL. Over-salting ruins the balance of the immune menu. J Clin Invest. 2015 Nov 2;125(11):4002-4.
58.Amara S, Tiriveedhi V. Inflammatory role of high salt level in tumor microenvironment (Review). Int J Oncol. 2017 May;50(5):1477-1481
59.Amara S, Alotaibi D, Tiriveedhi V. NFAT5/STAT3 interaction mediates synergism of high salt with IL-17 towards induction of VEGF-A expression in breast cancer cells. Oncol Lett. 2016 Aug;12(2):933-943
60.Amara S, Zheng M, Tiriveedhi V. Oleanolic acid inhibits high salt-induced exaggeration of warburg-like metabolism in breast cancer cells. Cell Biochem Biophys. 2016 Sep;74(3):427-34.
61.Amara S, Whalen M, Tiriveedhi V. High salt induces anti-inflammatory MΦ2-like phenotype in peripheral macrophages. Biochem Biophys Rep. 2016 Sep;7:1-9
62.Amara S, Ivy MT, Myles EL, Tiriveedhi V. Sodium channel γENaC mediates IL-17 synergized high salt induced inflammatory stress in breast cancer cells. Cell Immunol. 2016 Apr; 302:1-10
63.Davies RJ, Sandle GI, Thompson SM. Inhibition of the Na+,K(+)-ATPase pump during induction of experimental colon cancer. Cancer Biochem Biophys. 1991 Aug;12(2):81-94.
64.Thompson, Davies RJ. A high potassium diet prevents transepithelial depolarization in experimental colon cancer. In: Vitamins and Minerals in the Prevention and Treatment of Cancer, (Maryce M. Jacobs, Ed.), CRC Press, Boston, 1991, p 263.
65.Fine BP, Hansen KA, Walters TR, Denny TN. Dietary sodium deprivation inhibits cellular proliferation: evidence for circulating factor(s). In: Vitamins and Minerals in the Prevention and Treatment of Cancer, (Maryce M. Jacobs, Ed.), CRC Press, Boston, 1991, p 276.
66.Fine BP, Ponzio NM, Denny TN, Maher E, Walters TR. Restriction of tumor growth in mice by sodium-deficient diet. Cancer Res. 1988 Jun 15;48(12):3445-8.
67.Davies RJ, Daly JM. Potassium depletion and malignant transformation of villous adenomas of the colon and rectum. Cancer. 1984 Mar 15;53(6):1260-4.
68.Grivennikov SI, Greten FR, Karin M. Immunity, inflammation, and cancer. Cell. 2010 Mar 19;140(6):883-99.
69.Fernandes JV, Cobucci RN, Jatobá CA, Fernandes TA, de Azevedo JW, de Araújo JM. The role of the mediators of inflammation in cancer development. Pathol Oncol Res. 2015 Jul;21(3):527-34.
- Hagerling C, Casbon A J, Werb Z. Balancing the innate immune system in tumor development. Trends Cell Biol. 2015 Apr;25(4):214-20.
71.Wang K, Karin M. Tumor-elicited inflammation and colorectal cancer. Adv Cancer Res. 2015; 128:173-96.
72.Wang K, Karin M. The IL-23 to IL-17 cascade inflammation-related cancers. Clin Exp Rheumatol. 2015 Jul-Aug;33(4 Suppl 92): S87-90. Epub 2015 Oct 12.
73.Grivennikov SI, Wang K, Mucida D et al. Adenoma-linked barrier defects and microbial products drive IL-23/IL-17-mediated tumour growth. Nature. 2012 Nov 8;491(7423):254-8.
74.Kidane D, Chae WJ, Czochor J, Eckert KA, Glazer PM, Bothwell AL, Sweasy JB. Interplay between DNA repair and inflammation, and the link to cancer. Crit Rev Biochem Mol Biol. 2014 Mar-Apr;49(2):116-39.
75.Ioannidou A, Goulielmaki E, Garinis GA. DNA Damage: from chronic inflammation to age-related deterioration. Front Genet. 2016 Oct 25;7:187. eCollection 2016.
76.Dmitrieva NI, Burg MB. Analysis of DNA breaks, DNA damage response, and apoptosis produced by high NaCl. Am J Physiol Renal Physiol. 2008 Dec;295(6):F1678-88
77.Burg MB, Ferraris JD, Dmitrieva NI. Cellular response to hyperosmotic stresses. Physiol Rev. 2007 Oct;87(4):1441-74
78.Dmitrieva NI, Cai Q, Burg MB. Cells adapted to high NaCl have many DNA breaks and impaired DNA repair both in cell culture and in vivo. Proc Natl Acad Sci U S A. 2004 Feb 24;101(8):2317-22
79.Coussens LM, Zitvogel L, Palucka AK. Neutralizing tumor-promoting chronic inflammation: a magic bullet? Science. 2013 Jan 18;339(6117):286-91.
80.Coussens LM1, Werb Z. Inflammation and cancer. Nature. 2002 Dec 19-26;420(6917):860-7.
81.Yang T, Zhang A, Honeggar M, Kohan DE, Mizel D, Sanders K, Hoidal JR, Briggs JP, Schnermann JB. Hypertonic induction of COX-2 in collecting duct cells by reactive oxygen species of mitochondrial origin. J Biol Chem. 2005 Oct 14;280(41):34966-73
82.Zhang Z, Dmitrieva NI, Park JH, Levine RL, Burg MB. High urea and NaCl carbonylate proteins in renal cells in culture and in vivo, and high urea causes 8-oxoguanine lesions in their DNA. Proc Natl Acad Sci U S A. 2004 Jun 22;101(25):9491-6.
83.Zhou X, Ferraris JD, Burg MB. Mitochondrial reactive oxygen species contribute to high NaCl-induced activation of the transcription factor TonEBP/OREBP. Am J Physiol Renal Physiol. 2006 May;290(5):F1169-76
84.Zhou X, Ferraris JD, Cai Q, Agarwal A, Burg MB. Increased reactive oxygen species contribute to high NaCl-induced activation of the osmoregulatory transcription factor TonEBP/OREBP. Am J Physiol Renal Physiol. 2005 Aug;289(2):F377-85
85.Zou AP, Li N, Cowley AW Jr.Production and actions of superoxide in the renal medulla. Hypertension. 2001 Feb;37(2 Pt 2):547-53
86.Dornas WC1, de Lima WG, dos Santos RC, Guerra JF, de Souza MO, Silva M, Souza e Silva L, Diniz MF, Silva ME. High dietary salt decreases antioxidant defenses in the liver of fructose-fed insulin-resistant rats. J Nutr Biochem. 2013 Dec;24(12):2016-22.
87.Fellner RC, Cook AK, O’Connor PM, Zhang S, Pollock DM, Inscho EW. High-salt diet blunts renal autoregulation by a reactive oxygen species-dependent mechanism. Am J Physiol Renal Physiol. 2014 Jul 1;307(1):F33-40.
88.Jin K, Vaziri ND. Salt-sensitive hypertension in mitochondrial superoxide dismutase deficiency is associated with intra-renal oxidative stress and inflammation. Clin Exp Nephrol. 2014 Jun;18(3):445-52.
89.Ferrara N, Kerbel RS. Angiogenesis as a therapeutic target. Nature. 2005 Dec 15;438(7070):967-74
90.Wu P, Nielsen TE, Clausen MH. FDA-approved small-molecule kinase inhibitors. Trends Pharmacol Sci. 2015 Jul;36(7):422-39
91.Verheul HM, Pinedo HM. Inhibition of angiogenesis in cancer patients. Expert Opin Emerg Drugs. 2005 May;10(2):403-12
92.Takahashi S. Vascular endothelial growth factor (VEGF), VEGF receptors and their inhibitors for antiangiogenic tumor therapy. Biol Pharm Bull. 2011;34(12):1785-8
93.Ferrara N. Vascular endothelial growth factor: basic science and clinical progress. Endocr Rev. 2004 Aug;25(4):581-611
94.Pidgeon GP, Barr MP, Harmey JH, Foley DA, Bouchier-Hayes DJ. Vascular endothelial growth factor (VEGF) upregulates BCL-2 and inhibits apoptosis in human and murine mammary adenocarcinoma cells. Br J Cancer. 2001 Jul 20;85(2):273-8.
95.Liu F, Mu J, Yuan Z, Lian Q, Zheng S, Wu G, Liu E. Involvement of the lymphatic system in salt-sensitive hypertension in humans. Med Sci Monit. 2011 Oct;17(10):CR542-6.
96.Slagman MC, Kwakernaak AJ, Yazdani S, Laverman GD, van den Born J, Titze J, Navis G. Vascular endothelial growth factor C levels are modulated by dietary salt intake in proteinuric chronic kidney disease patients and in healthy subjects. Nephrol Dial Transplant. 2012 Mar;27(3):978-82.
97.Lankhorst S, Severs D, Markó L, Rakova N, Titze J, Müller DN, Danser AH, van den Meiracker AH. Salt sensitivity of angiogenesis inhibition-induced blood pressure rise: role of interstitial sodium accumulation? Hypertension. 2017 May;69(5):919-926.
98.Mizuno R, Isshiki M, Ono N, Nishimoto M, Fujita T. A high salt diet alters pressure-induced mechanical activity of the rat lymphatics with enhancement of myogenic characteristics. Lymphat Res Biol. 2015 Mar;13(1):2-9.
99.Yang GH, Zhou X, Ji WJ, Zeng S, Dong Y, Tian L, Bi Y, Guo ZZ, Gao F, Chen H, Jiang TM, Li YM. Overexpression of VEGF-C attenuates chronic high salt intake-induced left ventricular maladaptive remodeling in spontaneously hypertensive rats. Am J Physiol Heart Circ Physiol. 2014 Feb 15;306(4):H598-609.
100.Remo A, Simeone I, Pancione M, Parcesepe P, Finetti P, Cerulo L, Bensmail H, Birnbaum D, Van Laere SJ, Colantuoni V, Bonetti F, Bertucci F, Manfrin E, Ceccarelli M. Systems biology analysis reveals NFAT5 as a novel biomarker and master regulator of inflammatory breast cancer. J Transl Med. 2015 May 1;13:138. doi: 10.1186/s12967-015-0492-2.
- Warburg O, Wind F, Negelein E. The metabolism of tumors in the body. J Gen Physiol. 1927; 8:519-30.
102.Li C, Zhang G, Zhao L, Ma Z, Chen H. Metabolic reprogramming in cancer cells: glycolysis, glutaminolysis, and Bcl-2 proteins as novel therapeutic targets for cancer. World J Surg Oncol. 2016 Jan 20;14(1):15. doi: 10.1186/s12957-016-0769-9.
103.Xu W, Zhao X, Daha MR, van Kooten C. Reversible differentiation of pro- and anti-inflammatory macrophages. Mol Immunol. 2013 Mar;53(3):179-86
104.Zhang WC, Zheng XJ, Du L Jet al. High salt primes a specific activation state of macrophages, M(Na). Cell Res. 2015 Aug;25(8):893-910.
105.Brownlie RJ, Zamoyska R. T cell receptor signalling networks: branched, diversified and bounded Nat Rev Immunol. 2013 Apr;13(4):257-69.
106.Safa K, Ohori S, Borges TJ, Uehara M, Batal I, Shimizu T, Magee CN, Belizaire R, Abdi R, Wu C, Chandraker A, Riella LV. Salt accelerates allograft rejection through serum- and glucocorticoid-regulated kinase-1-dependent inhibition of regulatory t cells. J Am Soc Nephrol. 2015 Oct;26(10):2341-7
107.Hernandez AL, Kitz A, Wu C, Lowther DE, Rodriguez DM, Vudattu N, Deng S, Herold KC, Kuchroo VK, Kleinewietfeld M, Hafler DA. Sodium chloride inhibits the suppressive function of FOXP3+ regulatory T cells. J Clin Invest. 2015 Nov 2;125(11):4212-22
108.Bailey RL, Parker EA, Rhodes DG, Goldman JD, Clemens JC, Moshfegh AJ, Thuppal SV, Weaver CM. Estimating sodium and potassium intakes and their ratio in the american diet: data from the 2011-2012 NHANES. J Nutr. 2016 Mar 9. pii: jn221184. [Epub ahead of print]
109.Cordain, L, and Sebastian A. Unpublished data regarding the sodium and potassium content of 1,249 non-salted natural foods. 2017 – Found here.
110.Li Z, Langhans SA. Transcriptional regulators of Na, K-ATPase subunits. Front Cell Dev Biol. 2015 Oct 26;3:66. doi: 10.3389/fcell.2015.00066. eCollection 2015.
111.Clausen MV, Hilbers F, Poulsen H. The structure and function of the Na, K-atpase isoforms in health and disease. Front Physiol. 2017 Jun 6;8:371. doi: 10.3389/fphys.2017.00371. eCollection 2017.
112.Dmitrieva NI1, Cui K, Kitchaev DA, Zhao K, Burg MB. DNA double-strand breaks induced by high NaCl occur predominantly in gene deserts. Proc Natl Acad Sci U S A. 2011 Dec 20;108(51):20796-801.
113.Williams MF. Morphological evidence of marine adaptations in human kidneys. Med Hypotheses. 2006;66(2):247-57.
114.Sebastian A, Frassetto LA, Sellmeyer DE, Morris RC Jr. The evolution-informed optimal dietary potassium intake of human beings greatly exceeds current and recommended intakes. Semin Nephrol. 2006 Nov;26(6):447-53
115.Frassetto L, Morris RC Jr, Sellmeyer DE, Todd K, Sebastian A. Diet, evolution and aging–the pathophysiologic effects of the post-agricultural inversion of the potassium-to-sodium and base-to-chloride ratios in the human diet. Eur J Nutr. 2001 Oct;40(5):200-13
116.Cordain L, Eaton SB, Sebastian A, Mann N, Lindeberg S, Watkins BA, O’Keefe JH, Brand-Miller J. Origins and evolution of the western diet: Health implications for the 21st century. Am J Clin Nutr 2005;81:341-54.
117.Carrera-Bastos P, Fontes Villalba M, O’Keefe JH, Lindeberg S, Cordain L. The western diet and lifestyle and diseases of civilization. Res Rep Clin Cardiol 2011; 2: 215-235.
118.Dmitrieva NI, Burg MB. High NaCl promotes cellular senescence. Cell Cycle. 2007 Dec 15;6(24):3108-13.
119.Dmitrieva NI, Chen HT, Nussenzweig A, Burg MB. Knockout of Ku86 accelerates cellular senescence induced by high NaCl. Aging (Albany NY). 2009 Feb;1(2):245-53.
120.Yamakami Y, Yonekura R, Matsumoto Y, Takauji Y, Miki K, Fujii M, Ayusawa D.
High concentrations of NaCl induce cell swelling leading to senescence in human cells. Mol Cell Biochem. 2016 Jan;411(1-2):117-25.
121.Zhu H, Bhagatwala J, Pollock NK, Parikh S, Gutin B, Stallmann-Jorgensen I, Thomas J, Harshfield GA, Dong Y. High sodium intake is associated with short leukocyte telomere length in overweight and obese adolescents. Int J Obes (Lond). 2015 Aug;39(8):1249-53.
Loren Cordain, Ph.D.
As a professor at Colorado State University, Dr. Loren Cordain developed The Paleo Diet® through decades of research and collaboration with fellow scientists around the world.
More About The Author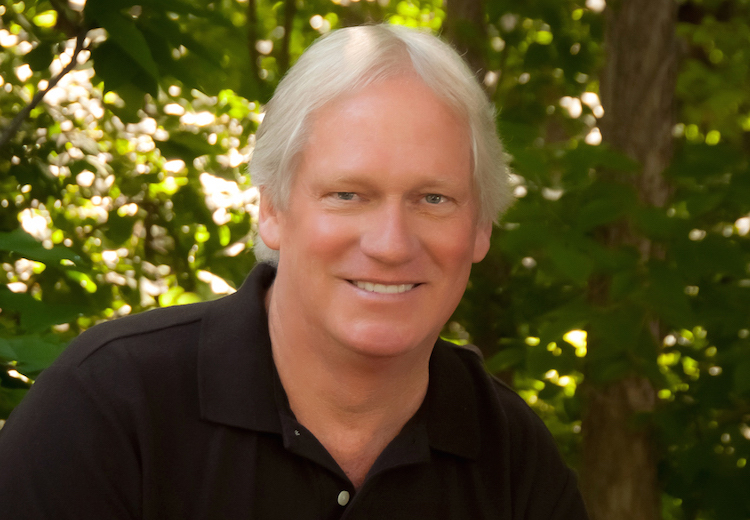