Why St. Louis Ribs Are So Healthy: Vitamin K2 Menaquinones
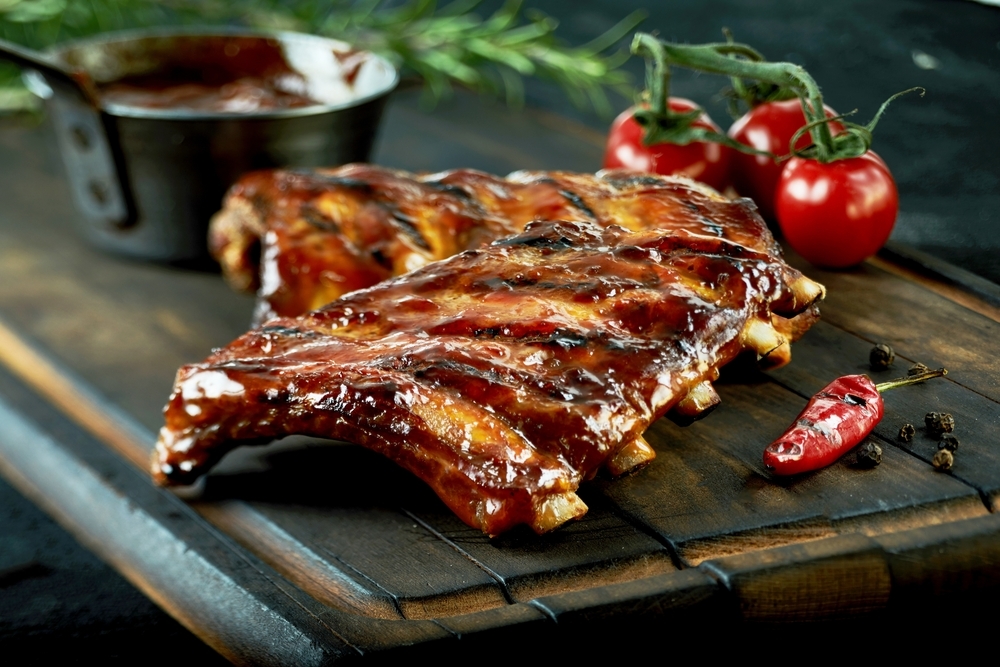
Introduction
Unless you are involved in the culinary arts or the meat industry, you probably haven’t given much thought to the term, “St. Louis Style Pork Ribs”. Is this dish some kind of specially spiced, spare rib recipe from St. Louis, MO? Did it originate in a colorful Saint Louis, a 20th century restaurant, or maybe it was first served at a St. Louis Cardinal baseball game in the 1930s? All three hypotheses seem to be plausible (1).
St. Louis Style Pork Ribs, first and foremost, represent a specific butchery cut of pork ribs recognized by the USDA (1). Figures 1 and 2 below demonstrate exactly how “St. Louis Style Pork Ribs” are butchered from hogs.
Figures 1 and 2. Butchery location of Saint Louis Style Pork Spare Ribs.
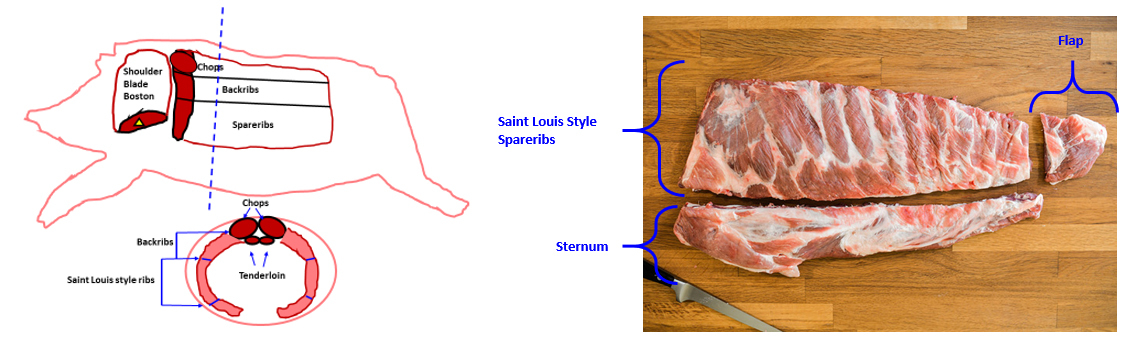
Because of their anatomical location slightly above pork bellies (the pork cut used for bacon manufacture), St. Louis Style Pork Spareribs represent the highest fat (combined muscle, bone, and fat) cut in the entire pig.
OK, so what is the big deal about St. Louis Style Pork Spareribs?
The official USDA position to prevent cardiovascular disease (CVD) is to eat less, not more saturated fat from fatty meats such as St. Louis Pork Spareribs.
But I believe there is some nutritional factor present in unadulterated, fatty pork meat, such as found in St. Louis Style Pork Spareribs that may not only prevent CVD, but may also prevent osteoporosis and be therapeutic for a wide variety of diseases which afflict the Western world.
Dietary Menaquinones (Vitamin K2)
St. Louis Style Pork Ribs have recently (2016) been discovered to be one of the richest dietary sources of menaquinones (2), also collectively known as vitamin K2. Ingestion of menaquinones may reduce the risk of cardiovascular disease (CVD) (3-10), osteoporosis (11-16), multiple sclerosis, and other autoimmune diseases (17-23) and cancer (24). The optimal intake of the ten dietary menaquinones (MK-4, MK-5, MK-6, MK-7, MK-8, MK-9, MK-10, MK-11, MK-12, and MK-13) is unknown (2, 25-27). Nevertheless, it is suspected that our current Western diet is low or deficient in total menaquinones (MK-4 to MK-13) [2, 25-27].
If you relish fatty cuts of pork (back ribs, spare ribs and St. Louis Style ribs), you are in luck, as the fattier the pork cuts, the more menaquinones they contain (2), which is a good thing for your bones (11-16), your heart (3-10) your immune system (17-23), and cancer prevention (24).
Table 1 demonstrates the concentrations of the ten menaquinones (MK-4 to MK-13) in both processed pork products (Kielbasa, Pork sausage, and Canadian bacon) and in fresh cuts of pork meat.
Table 1. The menaquinone content (μg/100 g) of processed and fresh pork meats (2), ND = not detected.
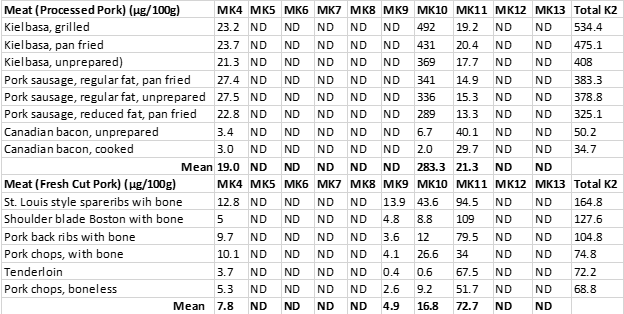
Processed pork meats showed a strong correlation (r = 0.94) between the total fat content of the various cuts and vitamin K content. This robust relationship is not surprising as vitamin K is a fat-soluble vitamin, and its hydrophobic (water avoiding) nature (30) explains why it is found in lower concentrations in leaner cuts of pork, which contain more water and less fat.
If you want to increase your dietary intake of menaquinones, you should eat fattier cuts of pork.
How about processed pork? Should you consider eating huge quantities of kielbasa, pork sausage links, and patties, and Canadian bacon to obtain your menaquinones? Probably not a good idea because all processed meats are highly salted.
Table 2 below demonstrates the sodium (Na+) and potassium (K+) contents of the processed pork meats from the study (2) and contrasts them to the fresh pork meats also tested in this study (2).
Despite being concentrated sources of menaquinones (Table 1), processed pork meat is also a concentrated source of Na+ with a 100 g serving to average 1152 mg of Na+ and also exhibiting an unhealthy K+/Na+ ratio of 0.31. For adults, the daily recommended intake of Na+ is 2,300 mg and for hypertensives, 1,500 mg or less (28). The Institute of Medicine (IOM) recommends a K+/Na+ ratio of 2.04, whereas contemporary Paleo diets maintain K+/Na+ ratios between 5.0 to 10.0 or higher (28, 29). Hence, for our stone-age ancestors, dietary K+/Na+ ratios below 1.0 (as present in processed pork meat) would have been impossible (28, 29).
In contrast, fresh cuts of fatty pork are not only rich sources of dietary menaquinones but also maintain healthful low Na+ concentrations together with high K+ concentrations (Table 2). Hence, fresh (unadulterated) pork, particularly fatty cuts represent excellent food choices to maintain high intakes of menaquinones while simultaneously keeping your Na+ intake low and K+ high.
Table 2. The sodium (Na+) and potassium (K+) contents of processed and fresh pork meats, along with their (Ca2+) and magnesium (Mg2+) contents.
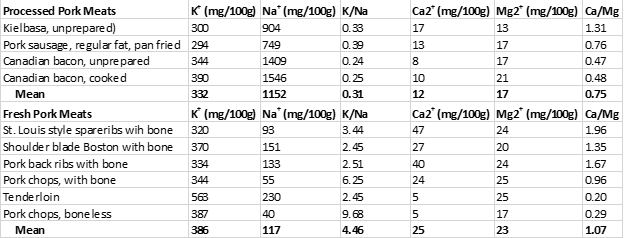
Other Dietary Sources of Menaquinones
Our Gut Bacteria
Vitamin K1, also known as phylloquinone, is the most abundant form of vitamin K in our diet and is found in highest concentrations in leafy, green vegetables. Our bodies have the capacity to convert K1 into menaquinones, but only into MK-4 (30). All other menaquinones (MK-5 to MK-13) are synthesized by bacteria and enter the human food chain either through dietary sources (particularly fermented foods) or from resident bacteria in our guts (25, 26, 30).
Cheese Products
Recently (2018-17), accurate menaquinone measurements have been reported for fermented dairy products, particularly cheeses (31, 32). Table 3 below shows the levels of menaquinones (MKs) in a variety of cheeses (31). As you can see from this table, a number of cheeses represent highly concentrated food sources of menaquinones, consumption of which would normally be a good thing from a nutritional perspective, as dietary menaquinones may reduce the risk of cardiovascular disease (CVD) (3-10), osteoporosis (11-16), multiple sclerosis and other autoimmune diseases (17-23) and cancer (24).
Unfortunately, as was the case with processed pork meats (Table 2), cheeses are almost universally high in salt, as can be seen from Table 4 below. All cheeses shown maintain K+/Na+ ratios considerably below 1.00, which represent impossible values from an evolutionary perspective (28, 29).
In addition to the impossible evolutionary K+/Na+ dietary ratios present in cheeses, cheeses also maintain impossible Ca2+/Mg2+ ratios, which could have never been consumed by ancestral humans before the advent of animal husbandry and dairy consumption (29, 33). Recommended Ca2+/Mg2+ ratios are about 2.00 (34), meaning that you should ingest about twice the amount of calcium relative to magnesium you obtain from your diet. Contrast this recommendation to the average Ca2+/Mg2+ ratio of 24.04 found in cheeses (Table 4) – meaning that cheeses contain ten times more calcium than magnesium, compared to recommended Ca2+/Mg2+ ratios (34).
Table 3. The menaquinone content (μg/100 g) of various cheeses (31), NM = not measured.
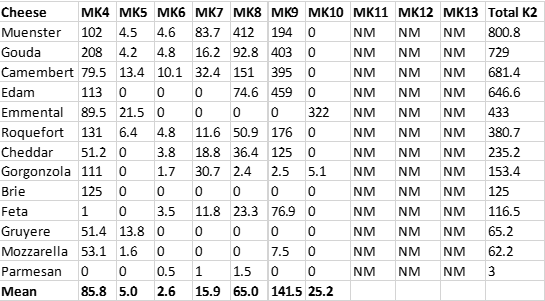
Table 4. The sodium (Na+) and potassium (K+) content of various cheeses, along with their (Ca2+) and magnesium (Mg2+) content.
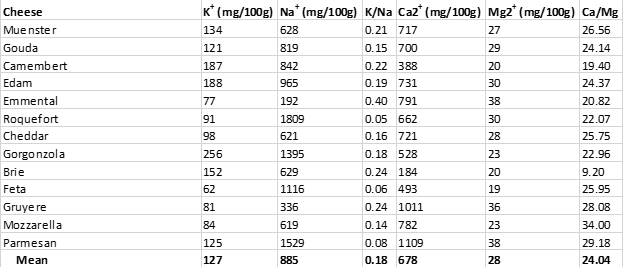
The Calcium-to-Magnesium Ratio
What are the health consequences of consuming enormous amounts of calcium relative to magnesium, even if you obtain the health-promoting dietary menaquinones found in fermented cheeses? And what are the adverse health consequences of consuming foods such as cheeses and processed meats loaded with salt and containing K+/Na+ ratios of less than 1.00?
High calcium intakes relative to low magnesium intakes, which frequently occur with high dairy consumption, are linked to an increased risk for cardiovascular disease (34-41) and osteoporosis (42-47). High salt diets increase your risk for hypertension, stroke, cardiovascular disease (28), autoimmune disease (48-57), gastric ulcers (58-63), all cancers (64-83), and ocular cataracts (84-92).
Let’s now take a step backward and show you the evolutionary basis for this seeming nutritional conundrum which requires us to obtain sufficient dietary menaquinones from high-fat foods but advises us against consuming high-fat salt laden cheeses and processed meats.
Where Were the Menaquinones in Our Ancient Diet?
John Speth, Ph.D., a world-renown anthropologist/archaeologist, has been my friend and scientific colleague for almost two decades. He recently published a paper that provides the nutrition and anthropology communities with a powerful insight into the evolutionary basis for menaquinones in the human diet (93).
Sir Arthur Conan Doyle stated, “There is nothing more deceptive than an obvious fact.” Indeed, in his paper (93), John has eloquently pointed out an obvious nutritional fact: Humans have been regularly consuming putrid, rotting, and fermented foods since the Middle Paleolithic era and likely earlier. This obvious fact had been apparently unknown to most anthropologists and nutritional scientists, including myself, until the publication of Dr. Speth’s paper in 2017.
In nature, the fermentation of fish, meat, fruits, and vegetables is haphazardly carried out by various bacterial species, particularly by lactic acid-producing bacteria (LAB) associated with modern food production including the following 11 bacterial genera: Carnobacterium, Enterococcus, Lactococcus, Lactobacillus, Leuconostoc, Oenococcus, Pediococcus, Streptococcus, Tetragenococcus, Vagococcus and Weisella (94-96). Many species within the LAB genera including Lactococcus lactis (subspecies. Cremoris) and Leuconostoc lactis synthesize high amounts of long-chain menaquinones (MK-8 to MK-10) (97). Other bacterial species which ferment Emmental and Jarlsberg cheese such as Propionibacterium freudenreichii produce high amounts of MK-9 (4H) (98). The bacteria (Bacillus subtilis) which ferments soybeans to make the Japanese food, natto produce unusually high concentrations of MK-7 (25).
All dietary menaquinones derived from the bacterial fermentation of various human foods, ultimately enrich our diet with long chain (MK-7 to MK-13) menaquinones, and have likely done so for millions of years (93).
Optimal dietary menaquinones intake has almost certainly been intimately linked to the consumption of fermented food since our genus, Homo’s, very beginnings more than 2 million years ago. Accordingly, these bacterially derived menaquinones have played an important evolutionary role in determining contemporary human nutritional requirements.
Summary and Practical Implications
Fatty pork cuts such as St. Louis Style Pork Ribs represent an excellent dietary choice for maximizing your intake of long-chain (MK-7 to MK-13) menaquinones intake without increasing your salt intake or compromising your nutritional K+/Na+ ratio. Consumption of long-chain dietary menaquinones reduces your risk for cardiovascular disease (CVD) (3-10), osteoporosis (11-16), multiple sclerosis, and other autoimmune diseases (17-23) and cancer (24).
In contrast, three other major sources of dietary menaquinones in the Western diet (cheese, processed pork meats, and fermented soy foods (soy sauce, miso, natto) contain excessive salt and calcium which yield evolutionarily impossible dietary K+/Na+ and Ca2+/Mg2+ ratios that increase the risk for CVD (34-41), osteoporosis (42-47), autoimmune disease (48-57), gastric ulcer (58-63) all cause cancer (64-83), ocular cataract (84-92).
The evolutionary basis for menaquinones in human nutrition comes not from recently introduced high salt processed meats, cheeses or soy products (all of which may be high in menaquinones), but rather from the evolutionary selective pressures derived from our species consumption of unadulterated bacterially fermented foods containing long-chain menaquinones for millions of years.
Looking to the Future
Although all forms of vitamin K (K1, K2, and vitamin K precursors [menadione]) have therapeutic effects, the longer chain vitamin K2 (particularly MK-7 to MK-13) appear to be more effective in ameliorating chronic disease (99, 100) than vitamin K1 (phylloquinone).
An alternative dietary strategy to maximize your intake of long-chain dietary menaquinones exists without increasing salt or calcium and unbalancing your K+/Na+ or Ca2+/Mg2+ dietary ratios. This strategy has been unknowingly suggested to extend the human lifespan and has shown to increase the lifespan of insects. More on this soon.
References
1. https://en.wikipedia.org/wiki/St._Louis-style_barbecue
2. Fu X, Shen X, Finnan EG, Haytowitz DB, Booth SL. Measurement of multiple vitamin k forms in processed and fresh-cut pork products in the U.S. food supply. J Agric Food Chem. 2016 Jun 8;64(22):4531-5.
3. Shea MK, Booth SL, Weiner DE, Brinkley TE, Kanaya AM, Murphy RA, Simonsick EM, Wassel CL, Vermeer C, Kritchevsky SB; Health ABC Study. Circulating Vitamin K is inversely associated with incident cardiovascular disease risk among those treated for hypertension in the health, aging, and body composition study (Health ABC). J Nutr. 2017 May;147(5):888-895
4. Shea MK, Booth SL, Miller ME, Burke GL, Chen H, Cushman M, Tracy RP, Kritchevsky SB. Association between circulating vitamin K1 and coronary calcium progression in community-dwelling adults: the Multi-Ethnic Study of Atherosclerosis. Am J Clin Nutr. 2013 Jul;98(1):197-208.
5. Shea MK, O’Donnell CJ, Hoffmann U, Dallal GE, Dawson-Hughes B, Ordovas JM, Price PA, Williamson MK, Booth SL. Vitamin K supplementation and progression of coronary artery calcium in older men and women. Am J Clin Nutr. 2009 Jun;89(6):1799-807
6. Gast GC, de Roos NM, Sluijs I, et al. A high menaquinone intake reduces the incidence of coronary heart disease. Nutr Metab Cardiovasc Dis. 2009 Sep;19(7):504-10.
7. Geleijnse JM, Vermeer C, Grobbee DE, Schurgers LJ, Knapen MH, van der Meer IM, Hofman A, Witteman JC. Dietary intake of menaquinone is associated with a reduced risk of coronary heart disease: the Rotterdam Study. J Nutr. 2004 Nov;134(11):3100-5.
8. Beulens JW, Bots ML, Atsma F, Bartelink ML, Prokop M, Geleijnse JM, Witteman JC, Grobbee DE, van der Schouw YT. High dietary menaquinone intake is associated with reduced coronary calcification. Atherosclerosis. 2009 Apr;203(2):489-93
9. Mayer O Jr, Seidlerová J, Vaněk J, Karnosová P, Bruthans J, Filipovský J, Wohlfahrt P, Cífková R, Windrichová J, Knapen MH, Drummen NE, Vermeer C. The abnormal status of uncarboxylated matrix Gla protein species represents an additional mortality risk in heart failure patients with vascular disease. Int J Cardiol. 2016 Jan 15;203: 916-22
10. Cundiff DK, Agutter PS. Cardiovascular disease death before age 65 in 168 countries correlated statistically with biometrics, socioeconomic status, tobacco, gender, exercise, macronutrients, and vitamin K. Cureus. 2016 Aug 24;8(8):e748. doi: 10.7759/cureus.748
11. Inaba N, Sato T, Yamashita T. Low-Dose daily intake of vitamin [C1] K (2) (menaquinone-7) improves osteocalcin γ-carboxylation: a double-blind, randomized controlled trials. J Nutr Sci Vitaminol (Tokyo). 2015;61(6):471-80
12. Shiraki M, Shiraki Y, Aoki C, Miura M. Vitamin K2 (menatetrenone) effectively prevents fractures and sustains lumbar bone mineral density in osteoporosis. J Bone Miner Res. 2000 Mar;15(3):515-21
13. Rønn SH, Harsløf T, Pedersen SB, Langdahl BL. Vitamin K2 (menaquinone-7) prevents age-related deterioration of trabecular bone microarchitecture at the tibia in postmenopausal women. Eur J Endocrinol. 2016 Dec;175(6):541-549
14. Knapen MH, Drummen NE, Smit E, Vermeer C & Theuwissen E. Three-year low-dose menaquinone-7 supplementation helps decrease bone loss in healthy postmenopausal women. Osteoporosis International 2013 24 2499–2507.
15. Kanellakis S, Moschonis G, Tenta R, Schaafsma A, van den Heuvel EG, Papaioannou N, Lyritis G & Manios Y. Changes in parameters of bone metabolism in postmenopausal women following a
12-month intervention period using dairy products enriched with calcium, vitamin D, and phylloquinone (vitamin K(1)) or menaquinone-7 (vitamin K (2)): the Postmenopausal Health Study II. Calcified Tissue International 2012 90 251–262.
16. Huang ZB, Wan SL, Lu YJ, Ning L, Liu C, Fan SW. Does vitamin K2 play a role in the prevention and treatment of osteoporosis for postmenopausal women: a meta-analysis of randomized controlled trials. Osteoporos Int. 2015 Mar;26(3):1175-86.
17. Riphagen IJ, Keyzer CA, Drummen NEA, de Borst MH, Beulens JWJ, Gansevoort RT, Geleijnse JM, Muskiet FAJ, Navis G, Visser ST, Vermeer C, Kema IP, Bakker SJL.
Prevalence and Effects of Functional Vitamin K Insufficiency: The PREVEND Study. Nutrients. 2017 Dec 8;9(12). pii: E1334. doi: 10.3390/nu9121334
18. Lasemi R1, Kundi M2, Moghadam NB3,4, Moshammer H1, Hainfellner JA5. Vitamin K2 in multiple sclerosis patients. Wien Klin Wochenschr. 2018 May;130(9-10):307-313.
19. Kusano J, Tanaka S, Matsuda H, et al. K1 and Vitamin K2 immunopharmacological effects on the peripheral lymphocytes of healthy subjects and dialysis patients, as estimated by the lymphocyte immunosuppressant sensitivity test. J Clin Pharm Ther. 2018 Jul 16. doi: 10.1111/jcpt.12747.
20. Myneni VD, Mezey E. Immunomodulatory effect of vitamin K2: Implications for bone health. Oral Dis. 2018 Mar;24(1-2):67-71.
21. Hatanaka H, Ishizawa H, Hirano T et al. Effects of vitamin K3 and K5 on proliferation, cytokine production, and regulatory T cell-frequency in human peripheral-blood mononuclear cells. Life Sci. 2014 Mar 18;99(1-2):61-8.
22. Meng K, Xu W, Hirano T et al. The effects of vitamin K1 and vitamin K2 on the proliferation, cytokine production and regulatory T-cell frequency in peripheral blood mononuclear cells of paediatric atopic dermatitis patients. Exp Dermatol. 2018 Apr 26. doi: 10.1111/exd.13671.
23. Wei FF, Thijs L, Zhang ZY et al. The risk of nephrolithiasis is causally related to inactive matrix Gla protein, a marker of vitamin K status: a Mendelian randomization study in a Flemish population. Nephrol Dial Transplant. 2018 Mar 1;33(3):514-522
24. Lamson DW, Plaza SM. The anticancer effects of vitamin K. Altern Med Rev. 2003 Aug;8(3):303-18
25. Walther B, Karl JP, Booth SL, Boyaval P. Menaquinones, bacteria, and the food supply: the relevance of dairy and fermented food products to vitamin K requirements. Adv Nutr. 2013 Jul 1;4(4):463-73
26. Beulens JW, Booth SL, van den Heuvel EG, Stoecklin E, Baka A, Vermeer C. The role of menaquinones (vitamin K₂) in human health. Br J Nutr. 2013 Oct;110(8):1357-68.
27. Shea MK, Booth SL. Concepts and controversies in evaluating vitamin k status in population-based studies. Nutrients. 2016 Jan 2;8(1). pii: E8. doi: 10.3390/nu8010008.
28. Sebastian A, Cordain L, Frassetto L, Banerjee T, Morris RC. Postulating the major environmental condition resulting in the expression of essential hypertension and its associated cardiovascular diseases: Dietary imprudence in daily selection of foods in respect of their potassium and sodium content resulting in oxidative stress-induced dysfunction of the vascular endothelium, vascular smooth muscle, and perivascular tissues. Med Hypotheses. 2018 Oct;119: 110-119
29. Cordain L. Nutritional characteristics of a contemporary diet based on Paleolithic food groups. J Am Nutraceutical Assoc 2002;5(3):15–24.
30. Shearer MJ, Okano T. key pathways and regulators of vitamin k function and intermediary metabolism. Annu Rev Nutr. 2018 Aug 21;38: 127-151
31. Vermeer C, Raes J, van ‘t Hoofd C, Knapen MHJ, Xanthoulea S. Menaquinone content of cheese. Nutrients. 2018 Apr 4;10(4). pii: E446. doi: 10.3390/nu10040446.
32. Fu X, Harshman SG, Shen X, Haytowitz DB, Karl JP, Wolfe BE, Booth SL. Multiple Vitamin K forms exist in dairy foods. Curr Dev Nutr. 2017 Jun 1;1(6):e000638. doi: 10.3945/cdn.117.000638.
33. Cordain L, Hickey MS, Kim K. Malaria and rickets represent selective forces for the convergent evolution of adult lactase persistence. Biodiversity in Agriculture: Domestication, Evolution, and Sustainability, edited by P. Gepts, T.R. Famula, R.L. Bettinger et al. Published by Cambridge University Press. # Cambridge University Press 2012.
34. Rosanoff A, Dai Q, Shapses SA. Essential nutrient interactions: does low or suboptimal magnesium status interact with vitamin d and/or calcium status? Adv Nutr. 2016 Jan 15;7(1):25-43
35. Varo P. Mineral element balance and coronary heart disease. Int J Vitam Nutr Res. 1974;44(2):267-73
36. Sato H, Takeuchi Y, Matsuda K, et al. Evaluation of the Predictive Value of the Serum Calcium-Magnesium Ratio for All-Cause and Cardiovascular Mortality in Incident Dialysis Patients. Cardiorenal Med. 2017 Dec;8(1):50-60
37. DiNicolantonio JJ, McCarty MF, O’Keefe JH. Decreased magnesium status may mediate the increased cardiovascular risk associated with calcium supplementation. Open Heart. 2017 May 22;4(1):e000617. doi: 10.1136/openhrt-2017-000617.
38. Park B, Kim MH, Cha CK, Lee YJ, Kim KC. High calcium-magnesium ratio in hair is associated with coronary artery calcification in middle-aged and elderly individuals. Biol Trace Elem Res. 2017 Sep;179(1):52-58
39. Kosch M, Hausberg M, Westermann G, et al. Alterations in calcium and magnesium content of red cell membranes in patients with primary hypertension. Am J Hypertens. 2001 Mar;14(3):254-8
40. Kisters K, Wessels F, Küper H, et al. Increased calcium and decreased magnesium concentrations and an increased calcium/magnesium ratio in spontaneously hypertensive rats versus Wistar-Kyoto rats: relation to arteriosclerosis. Am J Hypertens. 2004 Jan;17(1):59-62.
41. Resnick LM. Ionic basis of hypertension, insulin resistance, vascular disease, and related disorders. The mechanism of “syndrome X”. Am J Hypertens. 1993 Apr;6(4):123S-134S.
42. Tucker KL, Hannan MT, Chen H, Cupples LA, Wilson PW, Kiel DP. Potassium, magnesium, and fruit and vegetable intakes are associated with greater bone mineral density in elderly men and women. Am J Clin Nutr. 1999 Apr;69(4):727-36.
43. Ryder KM, Shorr RI, Bush AJ, Kritchevsky SB, Harris T, Stone K, Cauley J, Tylavsky FA. Magnesium intake from food and supplements is associated with bone mineral density in healthy older white subjects. J Am Geriatr Soc. 2005 Nov;53(11):1875-80
44. Stendig-Lindberg G1, Tepper R, Leichter I. Trabecular bone density in a two year controlled trial of peroral magnesium in osteoporosis. Magnes Res. 1993 Jun;6(2):155-63.
45. Rude RK, Singer FR, Gruber HE. Skeletal and hormonal effects of magnesium deficiency. J Am Coll Nutr. 2009 Apr;28(2):131-41
46. Sojka JE, Weaver CM. Magnesium supplementation and osteoporosis. Nutr Rev. 1995 Mar;53(3):71-4
47. New SA, Bolton-Smith C, Grubb DA, Reid DM. Nutritional influences on bone mineral density: a cross-sectional study in premenopausal women. Am J Clin Nutr. 1997 Jun;65(6):1831-9.
48. Wu C, Yosef N, Thalhamer T, Zhu C, Xiao S, Kishi Y, Regev A, Kuchroo VK. Induction of pathogenic TH17 cells by inducible salt-sensing kinase SGK1. Nature. 2013 Apr 25;496(7446):513-7.
49. Kleinewietfeld M, Manzel A, Titze J, Kvakan H, Yosef N, Linker RA, Muller DN, Hafler DA. Sodium chloride drives autoimmune disease by the induction of pathogenic TH17 cells. Nature. 2013 Apr 25;496(7446):518-22
50. Hucke S, Eschborn M, Liebmann M, Herold M, Freise N, Engbers A, Ehling P, Meuth SG, Roth J, Kuhlmann T, Wiendl H, Klotz L. Sodium chloride promotes pro-inflammatory macrophage polarization thereby aggravating CNS autoimmunity. J Autoimmun. 2016 Feb;67:90-101.
51. Zostawa J, Adamczyk J, Sowa P, Adamczyk-Sowa M. The influence of sodium on pathophysiology of multiple sclerosis. Neurol Sci. 2017 Mar;38(3):389-398.
52. Schatz V, Neubert P, Schröder A, Binger K, Gebhard M, Müller DN, Luft FC, Titze J, Jantsch J. Elementary immunology: Na+ as a regulator of immunity. Pediatr Nephrol. 2017 Feb;32(2):201-210.
53. Hernandez AL, Kitz A, Wu C, Lowther DE, Rodriguez DM, Vudattu N, Deng S, Herold KC, Kuchroo VK, Kleinewietfeld M, Hafler DA. Sodium chloride inhibits the suppressive function of FOXP3+ regulatory T cells. J Clin Invest. 2015 Nov 2;125(11):4212-22.
54. Yi B, Titze J, Rykova M, Feuerecker M, Vassilieva G, Nichiporuk I, Schelling G, Morukov B, Choukèr A. Effects of dietary salt levels on monocytic cells and immune responses in healthy human subjects: a longitudinal study. Transl Res. 2015 Jul;166(1):103-10.
55. Zhou X, Zhang L, Ji WJ, Yuan F, Guo ZZ, Pang B, Luo T, Liu X, Zhang WC, Jiang TM, Zhang Z, Li YM. Variation in dietary salt intake induces coordinated dynamics of monocyte subsets and monocyte-platelet aggregates in humans: implications in end organ inflammation. PLoS One. 2013 Apr 4;8(4):e60332.
56. Zhou X, Yuan F, Ji WJ, Guo ZZ, Zhang L, Lu RY, Liu X, Liu HM, Zhang WC, Jiang TM, Zhang Z, Li YM. High-salt intake induced visceral adipose tissue hypoxia and its association with circulating monocyte subsets in humans. Obesity (Silver Spring). 2014 Jun;22(6):1470-6.
57. Min B, Fairchild RL. Over-salting ruins the balance of the immune menu. J Clin Invest. 2015 Nov 2;125(11):4002-4.
58. Fox JG Dangler CA, Taylor NS, King A, Koh TJ, Wang TC. High-salt diet induces gastric epithelial hyperplasia and parietal cell loss, and enhances Helicobacter pylori colonization in C57BL/6 mice. Cancer Res. 1999 Oct 1;59(19):4823-8.
59. Shikata K, Kiyohara Y, Kubo M, Yonemoto K et al. A prospective study of dietary salt intake and gastric cancer incidence in a defined Japanese population: The Hisayama study. Int J Cancer. 2006 Jul 1;119(1):196-201.
60. Peleteiro B, Lopes C, Figueiredo C, Lunet N. Salt intake and gastric cancer risk according to Helicobacter pylori infection, smoking, tumour site and histological type. Br J Cancer. 2011 Jan 4;104(1):198-207.
61. Tsugane S. Salt, salted food intake, and risk of gastric cancer: epidemiologic evidence. Cancer Sci. 2005 Jan;96(1):1-6.
62. Wang XQ, Terry PD, Yan H. Review of salt consumption and stomach cancer risk: epidemiological and biological evidence. World J Gastroenterol. 2009 May 14;15(18):2204-13.
63. D’Elia L1, Galletti F, Strazzullo P. Dietary salt intake and risk of gastric cancer. Cancer Treat Res. 2014;159:83-95.
64. Amara S, Tiriveedhi V. Inflammatory role of high salt level in tumor microenvironment (Review). Int J Oncol. 2017 May;50(5):1477-1481
65. Amara S, Alotaibi D, Tiriveedhi V. NFAT5/STAT3 interaction mediates synergism of high salt with IL-17 towards induction of VEGF-A expression in breast cancer cells. Oncol Lett. 2016 Aug;12(2):933-943
66. Amara S, Zheng M, Tiriveedhi V. Oleanolic acid inhibits high salt-induced exaggeration of warburg-like metabolism in breast cancer cells. Cell Biochem Biophys. 2016 Sep;74(3):427-34.
67. Amara S, Whalen M, Tiriveedhi V. High salt induces anti-inflammatory MΦ2-like phenotype in peripheral macrophages. Biochem Biophys Rep. 2016 Sep; 7:1-9
68. Amara S, Ivy MT, Myles EL, Tiriveedhi V. Sodium channel γENaC mediates IL-17 synergized high salt induced inflammatory stress in breast cancer cells. Cell Immunol. 2016 Apr; 302:1-10
69. Amara S, Majors C, Roy B, Hill S, Rose KL, Myles EL, Tiriveedhi. Critical role of SIK3 in mediating high salt and IL-17 synergy leading to breast cancer cell proliferation. PLoS One. 2017 Jun 28;12(6):e0180097. doi: 10.1371/journal.pone.0180097
70. Jansson B. Potassium, sodium, and cancer: a review. J Environ Pathol Toxicol Oncol. 1996;15(2-4):65-73
71. Jansson B. Dietary, total body, and intracellular potassium-to-sodium ratios and their influence on cancer. Cancer Detect Prev. 1990;14(5):563-5
72. Jansson B. Intracellular electrolytes and their role in cancer etiology. In Thompson JR, Brown BW, eds. Cancer modeling. New York: Marcel Dekker 1987:1-59.
73. Jansson B. Geographic cancer risk and intracellular potassium/sodium ratios. Cancer Detect Prev. 1986;9(3-4):171-94
74. Jansson B, Jankovic J. Low cancer rates among patients with Parkinson’s disease. Ann Neurol. 1985 May;17(5):505-9
75. Newmark HL, Wargovich MJ, Bruce VR, Boynton AL, Kleine LP, Whitfield JF. Jansson B, Cameron IL. Ions and neoplastic development. In: Mastromarino AJ, Brattain MG, eds. Large bowel cancer. Clinical and basic science research. Cancer Research Monographs, Vol 3, New York: Praeger Publisher 1985:102-129.
76. Jansson B. Geographic mappings of colorectal cancer rates: a retrospect of studies, 1974-1984. Cancer Detect Prev. 1985;8(3):341-8
77. Jansson B. Seneca County, New York: an area with low cancer mortality rates. Cancer. 1981 Dec 1;48(11):2542-6
78. Jacobs MM, Pienta RJ. Relationships between potassium and cancer. In: Vitamins and Minerals in the Prevention and Treatment of Cancer (Jacobs MM [ed.]), 1991, CRC Press, Boston, Chapter 16, 227-245.
79. Davies RJ, Sandle GI, Thompson SM. Inhibition of the Na+,K(+)-ATPase pump during induction of experimental colon cancer. Cancer Biochem Biophys. 1991 Aug;12(2):81-94.
80. Thompson, Davies RJ. A high potassium diet prevents transepithelial depolarization in experimental colon cancer. In: Vitamins and Minerals in the Prevention and Treatment of Cancer, (Maryce M. Jacobs, Ed.), CRC Press, Boston, 1991, p 263.
81. Fine BP, Hansen KA, Walters TR, Denny TN. Dietary sodium deprivation inhibits cellular proliferation: evidence for circulating factor(s). In: Vitamins and Minerals in the Prevention and Treatment of Cancer, (Maryce M. Jacobs, Ed.), CRC Press, Boston, 1991, p 276.
82. Fine BP, Ponzio NM, Denny TN, Maher E, Walters TR. Restriction of tumor growth in mice by sodium-deficient diet. Cancer Res. 1988 Jun 15;48(12):3445-8.
83. Davies RJ, Daly JM. Potassium depletion and malignant transformation of villous adenomas of the colon and rectum. Cancer. 1984 Mar 15;53(6):1260-4.
84. CT Horng, YL Lee, HC Wu, HY et al. High salt diet induced the rapid myopic shift of cataract formation. Life Sci J, 2014;11:396-399.
85. Rodríguez-Sargent C, Cangiano JL, Berríos Cabán G, Marrero E, Martínez-Maldonado M. Cataracts and hypertension in salt-sensitive rats. A possible ion transport defect. Hypertension. 1987 Mar;9(3):304-8.
85. Donnelly CA, Seth J, Clayton RM, Phillips CI, Cuthbert J. Some plasma constituents correlate with human cataract location and nuclear colour. Ophthalmic Res. 1997;29(4):207-17.
86. Mathur G, Pai V. Comparison of serum sodium and potassium levels in patients with senile cataract and age-matched individuals without cataract. Indian J Ophthalmol. 2016 Jun;64(6):446-7.
87. Mirsamadi M, Nourmohammadi I, Imamian M. Comparative study of serum Na(+ )and K(+ ) levels in senile cataract patients and normal individuals. Int J Med Sci. 2004;1(3):165-169.
88. Bae JH, Shin DS, Lee SC, Hwang IC. Sodium intake and socioeconomic status as risk factors for development of age-related cataracts: The Korea National Health and Nutrition Examination Survey. PLoS One. 2015 Aug 19;10(8): e0136218. doi: 10.1371/journal.pone.0136218
89. Fossarello M, Orzalesi N, Corongiu FP, Biagini S, Casu M, Lai A.23Na NMR investigation of human lenses from patients with cataracts.FEBS Lett. 1985 May 20;184(2):245-8
90. Cumming RG, Mitchell P, Smith W. Dietary sodium intake and cataract: the Blue Mountains Eye Study. Am J Epidemiol. 2000 Mar 15;151(6):624-6
91. Rodríguez-Sargent C1, Berrios G, Irrizarry JE, Estapé ES, Cangiano JL, Martínez-Maldonado M. Prevention and reversal of cataracts in genetically hypertensive rats through sodium restriction. Invest Ophthalmol Vis Sci. 1989 Nov;30(11):2356-60.
92. Speth JD. Putrid meat and fish in the Eurasian Middle and Upper Paleolithic: Are we missing a key part of Neanderthal and Modern human diet? PaleoAnthropology 2017: 44−72. doi:10.4207/ PA.2017.ART 105
93. Mogensen G, Salminen S, O’Brien J. Food microorganisms – Health benefits, safety evaluation and strains with documented history of use in foods. Bull Int Dairy Fed, 2003;377:4-9.
94. De Vries MC, Vaughan EE, Kleerebezem M, Vos WM. Lactobacillus plantarum – survival, functional and potential probiotic properties in the human intestinal tract. Int Dairy J, 2006,16:1018-1028.
95. Azam M, Mohsin M, Ijaz H, Tulain UR et al. Lactic acid bacteria in traditional fermented Asian foods. Pak J Pharm Sci, 2017, 5:1803-1814.
96. Morishita T, Tamura N, Makino T, Kudo S. Production of menaquinones by lactic acid bacteria. J Dairy Sci. 1999 Sep;82(9):1897-903
97. Hojo K, Watanabe R, Mori T, Taketomo N. Quantitative measurement of tetrahydromenaquinone-9 in cheese fermented by Propionibacteria. J Dairy Sci. 2007 Sep;90(9):4078-83.
98. Gast GC, de Roos NM, Sluijs I, Bots ML, Beulens JW, Geleijnse JM, Witteman JC, Grobbee DE, Peeters PH, van der Schouw YT. A high menaquinone intake reduces the incidence of coronary heart disease. Nutr Metab Cardiovasc Dis. 2009 Sep;19(7):504-1
99. Cundiff DK, Agutter PS. Cardiovascular Disease Death Before Age 65 in 168 Countries Correlated Statistically with Biometrics, Socioeconomic Status, Tobacco, Gender, Exercise, Macronutrients, and Vitamin K. Cureus. 2016 Aug 24;8(8):e748. doi: 10.7759/cureus.748.
Loren Cordain, Ph.D.
As a professor at Colorado State University, Dr. Loren Cordain developed The Paleo Diet® through decades of research and collaboration with fellow scientists around the world.
More About The Author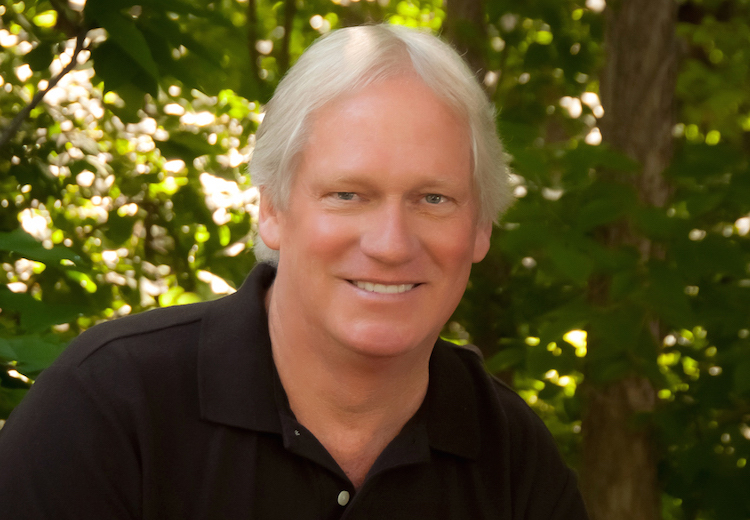