Brain Food: How to Fuel a Healthy Brain
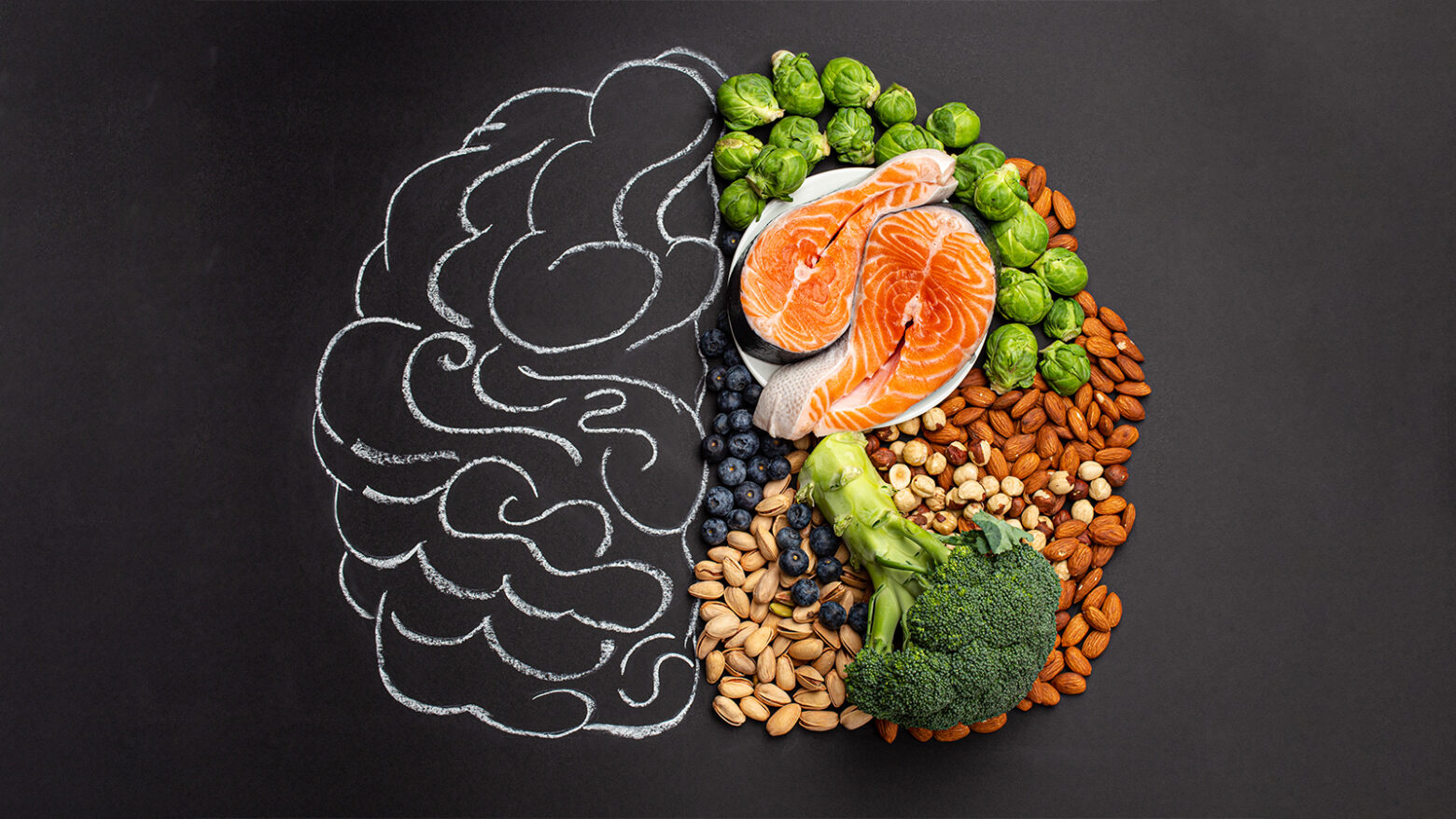
The human brain is amazingly complex. Even today, scientists have only the most rudimentary knowledge of how our brains work. Among the many questions yet to be answered, much attention is paid to which fuels are best: glucose or ketones? The answer isn’t straightforward.
Our knowledge of the brain is limited to anatomical structures and basic metabolic activity, as a result of our limited ability to access the brain. We have to use indirect measures such as electrochemical currents or oxygen flux.
The available tools help us understand cerebral metabolism, which is key to appropriately fueling a healthy brain. It’s also key to uncovering effective solutions for all manner of diseases, especially brain-based ones like Alzheimer’s, epilepsy, and Parkinson’s disease.
Before we dive into brain metabolism, let’s look to the past to better understand how our brains evolved.
The Evolution of the Human Brain
The human brain is relatively large among species. Scientists use the term “encephalization” to describe the size of an animal’s brain relative to its species’ expected size. It’s given as a quotient (EQ), with one being the average.
Humans have the highest EQ, between 7.4 and 7.8. The second largest EQ belongs to bottle nosed dolphins, at 5.3. [1] In absolute terms, only killer whales, blue whales, sperm whales, some species of dolphin, and African elephants have larger brains than us. So how is it that ours evolved to be so big? It’s still an open question, but there are some crucial clues.
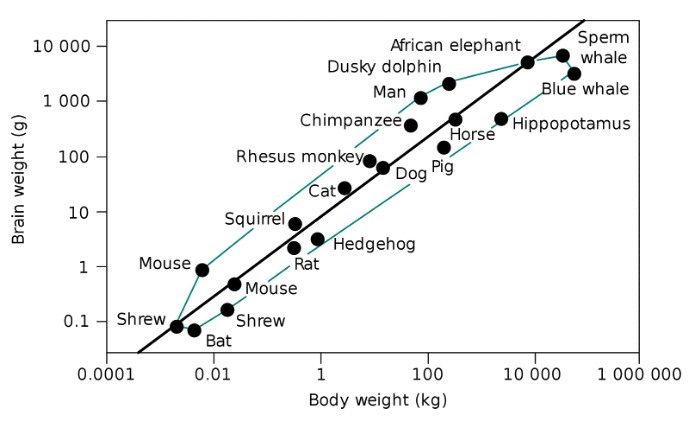
The brains of our evolutionary predecessors, Homo habilis, were about half the size of our human brains. Back then, man was already capable of using stone tools to scavenge the parts of prey that other animals couldn’t get access to, namely the brain and bone marrow. Our ancestors, thus, gained access to many more calories in this manner, especially fat. This started us on our path as “man the fat hunter.” [2] This was an early indicator that a growing brain and fat-based metabolism co-evolved.
With the advent of weaponry and pack hunting, our ancestors evolved into large-game specialists—hypercarnivores that consumed more than 70% of their calories from animals. This took place throughout most of the Stone Age some 2,600,000 to 11,000 years ago. [3]
This is also indicative of a fat-based metabolism. Others, however, think that the increase in calorie density could have mostly come from cooking tubers, which would be indicative of a more glucose-based metabolism. [4] However, this hypothesis appears unsupported by the timeline for the emergence of controlled fire use. [5]
The appearance of the human ZEB2 gene unlocked the evolution of a larger forebrain than in primates. [6] In other words, we got smarter. But, our expanding brains had higher energy needs. To accommodate this, our guts shrank and thus reduced their energy demand. They evolved into a digestive system better suited for nutrient and calorie-dense foods characteristic of a hypercarnivore’s diet. [7]
We have described some of the clues pertaining to how the brain grew so rapidly, evolutionarily speaking, as well as the evidence for the food that might have fueled it. Now, understanding brain energy economics will further help us understand whether we want to be running more of a fat/ketone-based metabolism or a glucose-based one.
The brain’s need for energy
A newborn’s brain is responsible for 60% of its basal (resting) metabolism, the majority of its energy needs. [8] This percentage decreases as the other organs grow. The average adult’s brain is responsible for approximately 20% of basal metabolism despite accounting for only about 2% of body mass.
The brain is always active. Its energy need is remarkably stable and independent of changes in mental or motor activity. The brain must get about 35% of its total energy from glucose. [9] The remaining 65% of energy can be supplied by ketones. Ketones can proportionately spare brain glucose utilization, and as more is spared, the deeper and longer a person is ketotic. [10]
On a standard American diet (or, simply, not on a ketogenic diet) our brains get close to 100% of their energy from glucose when awake.
As long as ketone production is sufficient, it has been experimentally demonstrated that there are no acute adverse symptoms when blood glucose drops to 9 mg/dL (0.5 mmol/L). [11] This is about 10 times lower than a healthy fasting blood sugar level.
By contrast, on a standard American diet (or, simply, not on a ketogenic diet) our brains get close to 100% of their energy from glucose when awake. [12] The glucose supplied to the brain comes from a mix of carbohydrates in the diet and gluconeogenesis.
Gluconeogenesis is your body’s way of making its own glucose using amino acids (protein) and glycerol (fat). Gluconeogenesis, in combination with our large fat stores, allows humans to live on a zero-carbohydrate diet—or simply fast for a few days, weeks or even a year or more—given enough body fat. [13] In the absence of dietary carbohydrates, the brain will get the glucose it needs from gluconeogenesis.
What fuels brain cells?
While at least 35% of the brain’s energy must come from glucose metabolized as pyruvate in mitochondria, it is still remarkably metabolically flexible. It can derive energy from multiple other sources including lactate, ketones, and fatty acids.
A brain that can efficiently switch between different fuel types is very desirable.
Pyruvate and lactate are products of glucose metabolism, and fatty acids can be turned into ketones. While glucose can supply all of the brain’s energy (ATP) needs, as already stated, ketones can supply about 65% and lactate can supply about 27%. [14]
Lactate is also a metabolic fulcrum—it shuttles between cells that produce it and cells that can consume it. [15] For example, during heavy exercise, the cells in the heart rely almost exclusively on lactate for fuel. The point here is that having a brain that can efficiently switch between different fuel types is very desirable.
So, what about brain cells? Brain cells can be grossly classified into neurons and astrocytes, at least for the outer layer of the brain (cortex). Astrocytes are a subtype of glial cells, as are microglia and oligodendrocytes. Which cells use which fuel is still a hotly debated question. It’s made harder to settle by the fact that virtually all neuroscience studies enroll subjects whose brains are consuming next to no ketones, thus skewing the evolutionary norm.
With that caveat in mind, the high-level view is that neurons are predominantly oxidative and astrocytes predominantly glycolytic. Oxidative cells produce energy using oxygen combined with any of the above fuels including the glucose end-products lactate or pyruvate, ketones, or acetate (a fatty acid). Glycolysis produces energy with or without oxygen using either of the glucose products (lactate or pyruvate).
The key takeaway is that the brain has many fuels available to it. In that way it is metabolically flexible, depending on its state. For example, in non-REM sleep, the brain burns about 5% more fat while blood ketone and fatty acid concentrations increase 20% and 50%, respectively. [16]
This metabolic flexibility is reflected in the brain’s multi-fuel use and its ability to seamlessly switch between glucose, ketones, and lactate.
Using diet to maintain a healthy brain
Pharmaceutical companies like Pfizer have stopped researching drugs for Alzheimer’s and Parkinson’s disease due to low profitability. [17] Drugs for these neurodegenerative diseases are only about half as successful (6.2%) as the drugs they produce for other conditions, whose success rate is already quite low (13.3%). [18]
Treatments for brain diseases also take 18% longer to develop, on average. The company’s failure isn’t surprising given their 1-drug-1-target approach to fix the most complex physiological system on the planet. However, there are other avenues to explore.
The diet supported by the most scientific evidence from human controlled trials to combat brain diseases is the ketogenic diet.
The diet supported by the most scientific evidence from human controlled trials to combat brain diseases is the ketogenic diet. No other diet has been tested as extensively or rigorously. Its beneficial effects on the brain include, but aren’t limited to, lowering the excitatory threshold (↓GABA/glutamate ratio), increasing cellular antioxidant capacity (↑astrocytes methylglyoxal detoxification), and normalizing the redox state (↑NAD/NADH). [19, 20, 21]
Think of the redox state like a battery’s ability to charge and provide energy to the machine it runs. Epilepsy has often been successfully treated with ketogenic diets for over a century, and since then the ketogenic diet’s application to brain conditions have grown to include traumatic brain injury, Alzheimer’s related memory issues, mood disorders, alcohol withdrawal, and more. [22, 23, 24, 25, 26]
The bottom line
Glucose fuels about one third of the brain’s energy needs; the rest can be fueled very well without it. Running a fat-based metabolism (i.e. using a keto diet) appears advantageous compared to a glucose metabolism, in terms of global redox status (“charge”), metabolic flexibility, energy efficiency, and oxidative stress buffering capacity.
Whether a more glucose-based metabolism from a non-ketogenic Paleo Diet can provide the same benefits remains an open question worth exploring.
Clues from our evolutionary past combined with recent evidence from research have improved our understanding of the brain’s metabolism and suggest a fat-based metabolism is likely beneficial to the brain.
What remains to be seen is the ideal diet for brain health and the prevention or treatment of brain-based diseases. Fortunately, the ketogenic diet and other previously under-researched interventions, many not mentioned here, are being investigated and showing great promise.
References
- Roth G, Dicke U. Evolution of the brain and intelligence. Trends in Cognitive Sciences [Internet]. 2005 [cited 14 April 2021];9(5):250-257. Available from: https://www.cell.com/trends/cognitive-sciences/fulltext/S1364-6613(05)00082-3?_returnURL=https%3A%2F%2Flinkinghub.elsevier.com%2Fretrieve%2Fpii%2FS1364661305000823%3Fshowall%3Dtrue
- Ben-Dor M, Gopher A, Hershkovitz I, Barkai R. Man the Fat Hunter: The Demise of Homo erectus and the Emergence of a New Hominin Lineage in the Middle Pleistocene (ca. 400 kyr) Levant. PLoS ONE [Internet]. 2011 [cited 14 April 2021];6(12):e28689. Available from: https://journals.plos.org/plosone/article?id=10.1371/journal.pone.0028689
- Ben‐Dor M, Sirtoli R, Barkai R. The evolution of the human trophic level during the Pleistocene. American Journal of Physical Anthropology [Internet]. 2021 [cited 14 April 2021]; Yearbook edition. Available from: https://onlinelibrary.wiley.com/doi/10.1002/ajpa.24247
- Aiello L. Brains and guts in human evolution: The Expensive Tissue Hypothesis. Brazilian Journal of Genetics [Internet]. 1997 [cited 14 April 2021];20(1):141-148. Available from: https://www.scielo.br/scielo.php?script=sci_arttext&pid=S0100-84551997000100023
- Wrangham R. Control of Fire in the Paleolithic: Evaluating the Cooking Hypothesis. Current Anthropology [Internet]. 2017 [cited 1 May 2021];58(S16):S303-S313. Available from: https://www.journals.uchicago.edu/doi/10.1086/692113
- Benito-Kwiecinski S, Giandomenico S, Sutcliffe M, Riis E, Freire-Pritchett P, Kelava I et al. An early cell shape transition drives evolutionary expansion of the human forebrain. Cell [Internet]. 2021 [cited 14 April 2021];. Available from: https://www.cell.com/cell/fulltext/S0092-8674(21)00239-7
- Aiello L, Bates N, Joffe T. In defense of the Expensive Tissue Hypothesis. Evolutionary Anatomy of the Primate Cerebral Cortex [Internet]. 2001 [cited 14 April 2021];57-78. Available from: https://www.cambridge.org/core/books/evolutionary-anatomy-of-the-primate-cerebral-cortex/in-defense-of-the-expensive-tissue-hypothesis/1FAF31D65717854AD7F4F04CD97350F8
- Raichle M, Gusnard D. Appraising the brain’s energy budget. Proceedings of the National Academy of Sciences [Internet]. 2002 [cited 14 April 2021];99(16):10237-10239. Available from: https://www.pnas.org/content/99/16/10237.full
- Cahill G. Fuel Metabolism in Starvation. Annual Review of Nutrition [Internet]. 2006 [cited 14 April 2021];26(1):1-22. Available from: https://www.annualreviews.org/doi/10.1146/annurev.nutr.26.061505.111258?url_ver=Z39.88-2003&rfr_id=ori%3Arid%3Acrossref.org&rfr_dat=cr_pub++0pubmed
- Zhang Y, Kuang Y, Xu K, Harris D, Lee Z, LaManna J et al. Ketosis Proportionately Spares Glucose Utilization in Brain. Journal of Cerebral Blood Flow & Metabolism [Internet]. 2013 [cited 14 April 2021];33(8):1307-1311. Available from: https://www.ncbi.nlm.nih.gov/pmc/articles/PMC3734783/
- Drenick E, Alvarez L, Tamasi G, Brickman A. Resistance to Symptomatic Insulin Reactions after Fasting. Journal of Clinical Investigation [Internet]. 1972 [cited 14 April 2021];51(10):2757-2762. Available from: https://www.ncbi.nlm.nih.gov/pmc/articles/PMC332976/?tool=pmcentrez&report=abstract
- Cahill Jr G. F., Owen O. E. Starvation and survival. Transactions of the American Clinical and Climatological Association [Internet]. 1968 [cited 14 April 2021];79: 13–20. Available from: https://www.ncbi.nlm.nih.gov/pmc/articles/PMC2441175/
- Stewart W, Fleming L. Features of a successful therapeutic fast of 382 days’ duration. Postgraduate Medical Journal [Internet]. 1973;49(569):203-209. Available from: https://www.ncbi.nlm.nih.gov/pmc/articles/PMC2495396/
- van Hall G, Stømstad M, Rasmussen P, Jans Ø, Zaar M, Gam C et al. Blood Lactate is an Important Energy Source for the Human Brain. Journal of Cerebral Blood Flow & Metabolism [Internet]. 2009 [cited 14 April 2021];29(6):1121-1129. Available from: https://journals.sagepub.com/doi/10.1038/jcbfm.2009.35
- Brooks G. Lactate as a fulcrum of metabolism. Redox Biology [Internet]. 2020 [cited 14 April 2021];35:101454. Available from: https://www.sciencedirect.com/science/article/pii/S2213231720300422?via%3Dihub
- Aalling N, Nedergaard M, DiNuzzo M. Cerebral Metabolic Changes During Sleep. Current Neurology and Neuroscience Reports [Internet]. 2018 [cited 14 April 2021];18(9). Available from: https://link.springer.com/article/10.1007/s11910-018-0868-9
- Dwyer C. Pfizer Halts Research Into Alzheimer’s And Parkinson’s Treatments [Internet]. NPR.org. 2018 [cited 14 April 2021]. Available from: https://www.npr.org/sections/thetwo-way/2018/01/08/576443442/pfizer-halts-research-efforts-into-alzheimers-and-parkinsons-treatments
- Peters S. CNS Drugs Take Longer to Develop and Have Lower Success Rates than Other Drugs [Internet]. Tufts Center for the Study of Drug Development. 2021 [cited 14 April 2021]. Available from: https://static1.squarespace.com/static/5a9eb0c8e2ccd1158288d8dc/t/5aa2bf604192023932fe1561/1520615264660/PR-NOVDEC14.pdf
- Xin L, Ipek Ö, Beaumont M, Shevlyakova M, Christinat N, Masoodi M et al. Nutritional Ketosis Increases NAD+/NADH Ratio in Healthy Human Brain: An in Vivo Study by 31P-MRS. Frontiers in Nutrition [Internet]. 2018 [cited 14 April 2021];5. Available from: https://www.frontiersin.org/articles/10.3389/fnut.2018.00062/full
- Jarrett S, Milder J, Liang L, Patel M. The ketogenic diet increases mitochondrial glutathione levels. Journal of Neurochemistry [Internet]. 2008 [cited 14 April 2021];106(3):1044-1051. Available from: https://onlinelibrary.wiley.com/doi/full/10.1111/j.1471-4159.2008.05460.x
- Magistretti P, Allaman I. A Cellular Perspective on Brain Energy Metabolism and Functional Imaging. Neuron [Internet]. 2015 [cited 14 April 2021];86(4):883-901. Available from: https://www.cell.com/neuron/fulltext/S0896-6273(15)00259-7?_returnURL=https%3A%2F%2Flinkinghub.elsevier.com%2Fretrieve%2Fpii%2FS0896627315002597%3Fshowall%3Dtrue
- McDougall A, Bayley M, Munce S. The ketogenic diet as a treatment for traumatic brain injury: a scoping review. Brain Injury [Internet]. 2018 [cited 14 April 2021];32(4):416-422. Available from: https://www.tandfonline.com/doi/abs/10.1080/02699052.2018.1429025?journalCode=ibij20
- Ota M, Matsuo J, Ishida I, Takano H, Yokoi Y, Hori H et al. Effects of a medium-chain triglyceride-based ketogenic formula on cognitive function in patients with mild-to-moderate Alzheimer’s disease. Neuroscience Letters [Internet]. 2019 [cited 14 April 2021];690:232-236. Available from: https://www.sciencedirect.com/science/article/pii/S0304394018307304?via%3Dihub
- 13. IJff D, Postulart D, Lambrechts D, Majoie M, de Kinderen R, Hendriksen J et al. Cognitive and behavioral impact of the ketogenic diet in children and adolescents with refractory epilepsy: A randomized controlled trial. Epilepsy & Behavior [Internet]. 2016 [cited 14 April 2021];60:153-157. Available from: https://www.epilepsybehavior.com/article/S1525-5050(16)30055-5/fulltext
- White H, Venkatesh B, Jones M, Kruger P, Walsham J, Fuentes H. Inducing ketogenesis via an enteral formulation in patients with acute brain injury: a phase II study. Neurological Research [Internet]. 2020 [cited 14 April 2021];42(4):275-285. Available from: https://www.tandfonline.com/doi/abs/10.1080/01616412.2019.1709743?journalCode=yner20
- Wiers C, Vendruscolo L, van der Veen J, Manza P, Shokri-Kojori E, Kroll D et al. Ketogenic diet reduces alcohol withdrawal symptoms in humans and alcohol intake in rodents. Science Advances [Internet]. 2021 [cited 14 April 2021];7(15):eabf6780. Available from: https://advances.sciencemag.org/content/7/15/eabf6780/tab-pdf
Raphael Sirtoli, M.Sc.
Raphael Sirtoli has an M.Sc. in molecular biology and is a Ph.D. candidate in health sciences at the Behavioral and Molecular Lab in Portugal. Raphael is also co-founded Nutrita.
More About The Author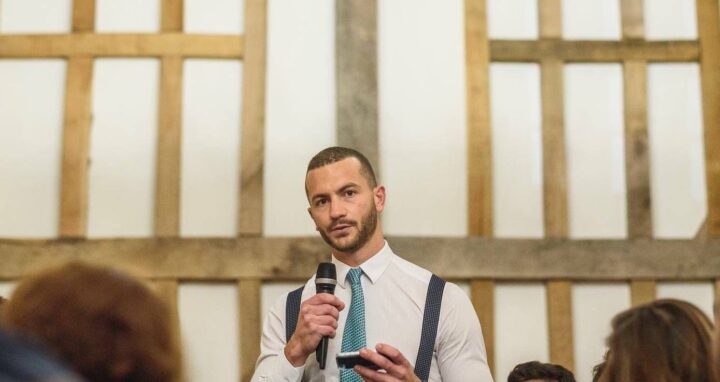